Coverage: Russian Submarine-Tracking Satellite heads for Destructive Re-Entry
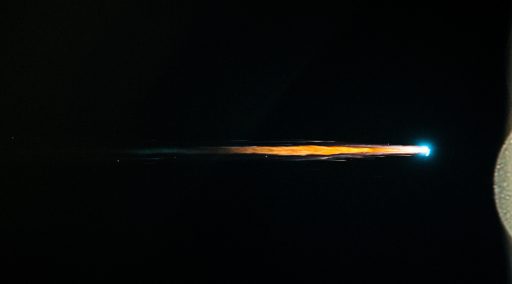
The Russian Volga Upper Stage and Kanopus ST submarine-tracking satellite are rapidly approaching an untargeted destructive re-entry after completing a significant orbit-lowering maneuver in response to the failed separation of the satellite following its arrival in a 690-Kilometer orbit on Saturday.
Considered a total loss, the Kanopus ST – Volga Complex made a significant orbit-lowering maneuver in the early hours on Sunday, setting the craft up for destructive re-entry, likely to take place on Tuesday. Considering the mass and construction of the spacecraft, some components may survive re-entry and make it to the ground.
Live Updates: Kanopus ST & Volga approach fiery Re-Entry
.
Live Satellite Tracker
Kanopus ST fails to Separate
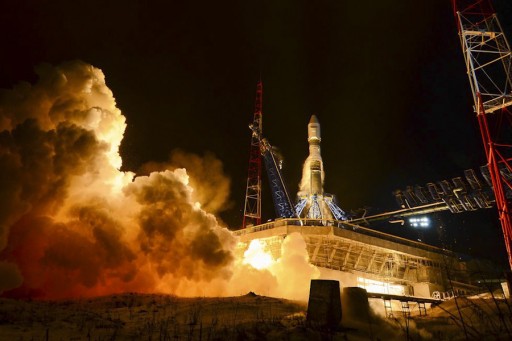
The Kanopus ST Satellite, along with a radar calibration sphere as secondary payload, blasted off atop a Soyuz 2-1v rocket at 14:09 UTC on Saturday from Site 43/4 at the Plesetsk Cosmodrome, Russia’s primary military launch site. The Soyuz 2-1v rocket ascended from the snow-covered launch base under the loud thunder of its NK-33 and RD-0110R engines that lifted the rocket with a total thrust of 188 metric ton-force. The 157,000-Kilogram vehicle swung to the north in order to head to a high-inclination orbit, firing the first stage for a little over two minutes before completing the typical hot-staging sequence – marking the successful return to flight of the NK-33 engine after 2014’s Antares launch failure. The second stage burned its RD-0124 engine for a little under four and a half minutes to boost the stack into orbit.
The Volga upper stage was separated around seven minutes into the mission to assume control of the flight for two main engine burns. The first of the burns boosted the stack into an elliptical orbit and was followed by a coast phase to carry the stack to the apogee of the orbit for the circularization maneuver. A successful spacecraft separation was confirmed a little under two hours after launch and the Russian Ministry of Defence confirmed that data from the Kanopus ST satellite was being received.
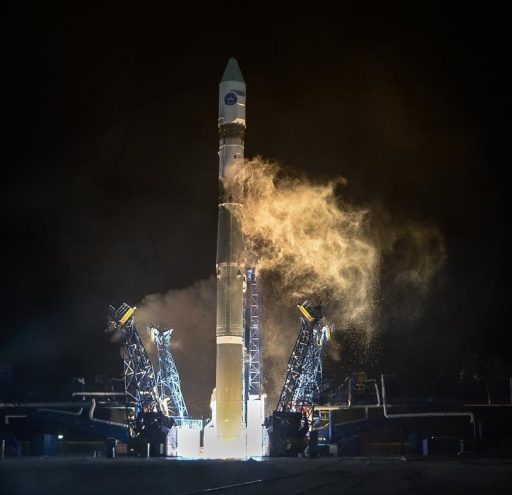
The second stage of the Soyuz 2-1v rocket was tracked in the following orbit:
2015-072C - 208.4 x 681.4 km - 98.19°
Prior to launch, information came forward that the Kanopus ST satellite was targeting an orbit of 702 Kilometers inclined 98°, however only two objects showed up in that orbit instead of the expected three (Kanopus ST, КЮА 1 calibration sphere, Volga Upper Stage):
2015-071A - 685.0 x 694.8 km - 98.18° 2015-072B - 684.5 x 693.7 km - 98.18°
Media reports emerged on Sunday indicating that one of the two satellites failed to separate from the Volga upper stage, not immediately identifying which of the two satellites had remained attached to the upper stage. Later on Sunday, it became clear that it was indeed Kanopus ST that had not separated, reportedly due to one of four pyrotechnic separation devices not operating as expected. The two objects delivered to Sun Synchronous Orbit in this launch were assigned designations of Kosmos 2511 (Kanopus ST – Volga Complex) and Kosmos 2512 (КЮА 1 that had separated nominally from the upper stage.
Kanopus ST Orbit Reduction Maneuver
On Sunday, between 1 and 10 UTC, likely around 2 UTC, the Kanopus ST – Volga Complex conducted a significant orbit reduction maneuver, lowering its perigee to accelerate the orbital decay from several years in the previous orbit to a few days in the short-lived elliptical orbit. Tracking data released on Sunday showed the stack in the following orbit:
Kosmos 2511 - 113.5 x 591.4 km - Inc: 98.17° - Period: 91.59 min
This maneuver took place around ten hours after the failed separation of the primary payload, likely conducted by the Volga Upper Stage as a maneuver initially intended to dispose of the Upper Stage. The extra mass of the attached Kanopus satellite meant that the stage did not have enough propellant reserves to completely deorbit the stack and was only able to bring down the perigee to an altitude where it skims the upper reaches of the atmosphere for a rapid decay of the orbit.
.
Kanopus ST Satellite
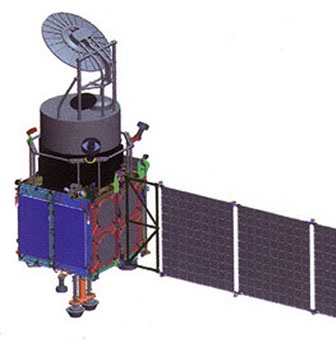
The Kanopus-ST satellite is a small remote sensing spacecraft. It enters a program that is operated in a dual-use fashion, delivering data for civilian and military applications.
According to documentation, Kanopus ST hosts a Microwave radiometer with a conical scanning geometry achieving a swath width of 2,200 Kilometers and a resolution of 12 to 160 Kilometers, and a multi-spectral imaging system covering the visible wavelengths across a 1,000-Kilometer swath, reaching a spatial resolution of 30 to 50 meters.
Comments made by Russian Military Officials in 2010 indicated that the Kanopus ST satellite would be capable of scanning the underwater areas for submarines, however, the published specifications would not permit such a detection. The relative lack of information on the satellite’s payloads and the secrecy surrounding its launch may suggest a more military-oriented role of the mission.
.
Re-Entry Prediction & Associated Risks
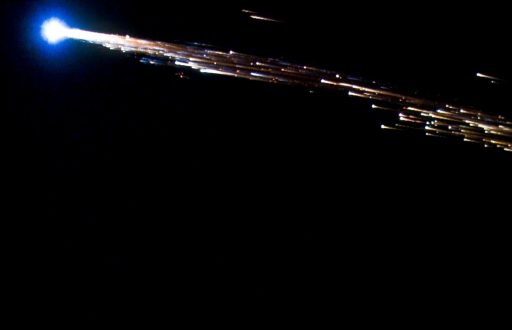
Objects drop out of orbit and re-enter Earth’s atmosphere every day, however, the re-entry of large objects with masses of several metric tons is rather rare, coming about once every year or two.
International agreements have been made in recent years to ensure heavy objects are deorbited in a controlled fashion to avoid risk to the public, but there are are many old, heavy satellites still to re-enter and accidents such as the recent Progress mishap and Saturday’s partially failed launch can leave large spacecraft in short-lived orbits.
To date, there has not been a casualty reported in association with a re-entering man-made object. But there have been cases of re-entries occurring over or near populated areas and remnants of spacecraft are known to survive re-entry. There have been cases of objects from spacecraft being recovered on land or washed ashore if re-entry occurred over the Ocean.
A rule of thumb often used when it comes of the estimation of an uncontrolled satellite re-entering over land is the distribution of land and ocean on the surface of the Earth. 75% of the Earth is covered by water so often a probability of 25% of a satellite hitting land can be found in the media.
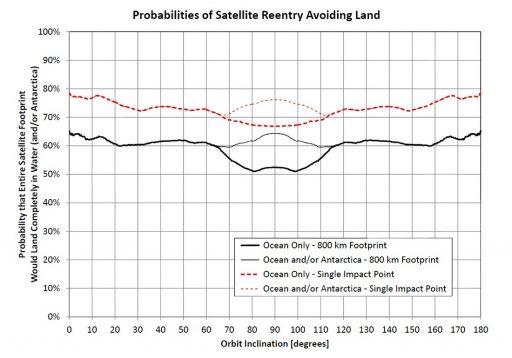
However, the matter becomes more complicated when taking into account the orbital inclination of the satellite that drives the fraction of an orbit spent over land given Earth’s uneven distribution of land as a function of latitude.
In a study published by M. Matney in ‘Orbital Debris Quarterly’ the probabilities of satellite re-entry avoiding land was calculated for all orbital inclinations from equatorial to polar orbits. The study evaluated a hypothetical satellite re-entry with a debris footprint of 800 Kilometers in the along-track direction, but no width, or cross track dispersion to simplify the model.
The Kanopus ST – Volga Stack orbits Earth in a polar orbit at an inclination of 98 degrees which means that debris could fall virtually anywhere on the planet, covering all inhabited areas of the planet, but also passing over the vast areas of the Ocean. It has been calculated that the probability of debris ending up on land for this type of orbit is approximately 49%.
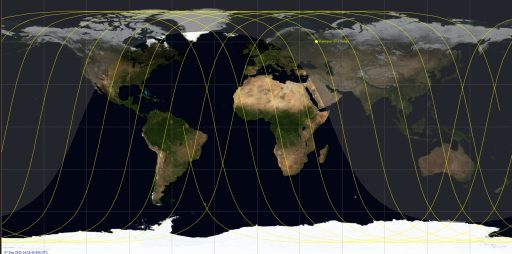
Through tracking of the spacecraft and orbit propagation, it is possible to estimate the time and possible location of re-entry in advance, but this calculation is strongly dependent on a number of factors that can not be accurately determined. These factors include the conditions of the atmosphere that drive the speed of orbital decay and are influenced by solar activity that can not be predicted with 100% accuracy. Also, the orientation and attitude rate of the spacecraft plays a major role in the speed of its orbital decay as they influence the drag experienced by the craft.
Any re-entry prediction is *always* associated with a window of uncertainty given the unknowns described above. Many media outlets will give the re-entry time and even locations as absolute values, but the truth is that when re-entry is still several days away, the error bar on any prediction can be 24 hours in either direction or more. Even on the day of re-entry, calculations can only narrow down the approximate time and location to within about one orbit of Earth so that areas of risk can be identified and other areas can be excluded.
.
The Re-Entry Process & Surviving Components
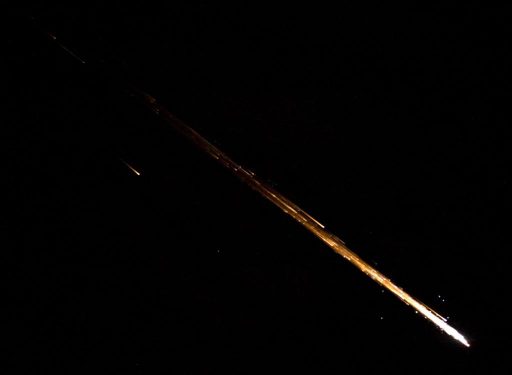
Initially, Kanopus ST began losing altitude slowly caused by drag in the upper atmosphere in the form of collisions of the spacecraft with ions and molecules present in the upper reaches of the atmosphere leading to a loss of velocity on the spacecraft that causes the orbital altitude to drop.
As the spacecraft enters a lower and lower orbit, drag increases and the speed of orbital decay picks up. In some cases, spacecraft can enter a tumble in the days leading up to entry, but in many cases the craft will enter an aerodynamically stable attitude in the tenuous upper layers of the atmosphere.
The speed of orbital decay will depend on a number of factors, not all of which can be known precisely such as the state of Earth’s atmosphere that can only be estimated based on current solar weather using the 10.7cm radio flux, Kp-Index and space weather forecast models to feed decay simulations. Also, the spacecraft attitude plays a role in the level of drag experienced by the vehicle. Therefore, decay predictions are always associated with an error bar that corresponds to about 20% of the time from the prediction to the predicted decay time.
Over its final hours in orbit, the craft will be tracked by ground-based radars to precisely determine its orbit. The United States operate a tracking system that provides data to the public to allow an independent calculation of the re-entry time.
When re-entry comes, its position can be calculated from orbital data gathered in the last orbits ahead of the event, but the most precise data will be provided through space-based assets of the U.S. military that can track the signature of spacecraft re-entries and so pin-point their timing and location with high precision.
As the spacecraft drops in altitude, it will approach the dense layers of the atmosphere for its steep plunge. The exact timing of orbital decay depends on the current state of the atmosphere which is known to expand and contract as a result of solar activity. Once hitting the dense atmosphere, Kanopus/Volga will start feeling the effects of re-entry.
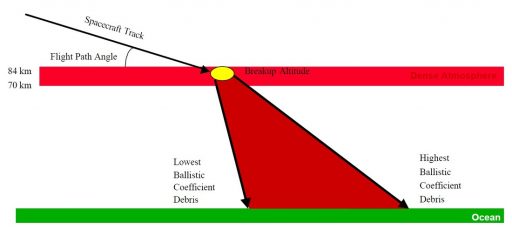
The onset of re-entry usually occurs at an altitude of 120 to 100 Kilometers when the spacecraft enters the dense atmosphere, initially not slowing down at a fast rate yet but already interacting with plenty of molecules in the upper reaches of the atmosphere. Given the high speed of the object, traveling at 7.7 Kilometers per second, air in front of the craft is compressed creating a shock wave layer in which some of the molecules are separated into ions, creating the typical visible plasma layer that can be present even minutes before disintegration starts.
The Entry Point, as defined by USSTRATCOM and represented in all their data, refers to the spacecraft passing 80 Kilometers in altitude where drag builds up to a destructive force, triggering the onset of the disintegration of the spacecraft structure. The shock wave layer forming just in front of the spacecraft and any separated components leads to considerable heating that causes the incineration of the majority of the spacecraft structure. The mechanical deceleration experienced during re-entry can be up to 20Gs further crushing the structural components and causing the break-up of the spacecraft.
The mechanical energy of a re-entering satellite is ~3.2 x 10^7 J/kg which would be sufficient to easily vaporize the entire satellite if all of this energy were converted into heat entirely absorbed by the satellite structure. However, only a fraction of a satellite’s total energy is converted into heat absorbed by its body and depends on a number of factors such as flight path angle and velocity as well as the properties of the object (shape, area and mass).
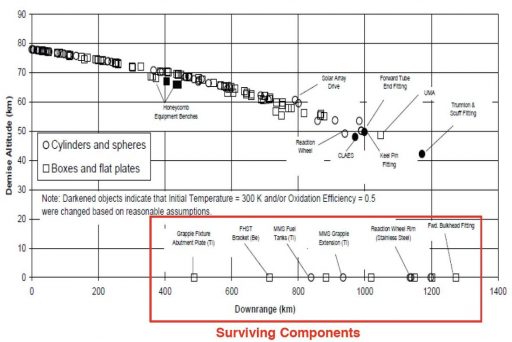
Generally, loose components with a high area to mass ratio such as solar arrays and antenna reflectors are lost first, becoming detached from a satellite at an altitude around 100 Kilometers. The main spacecraft body usually experiences disintegration at an altitude of 68 to 88 Kilometers due to the heat and dynamic loads experienced during entry.
However, it is known that about 20 to 40% of a re-entering satellite’s total mass reach Earth’s surface. It has to be considered that the spacecraft and any surviving components travel at a high horizontal velocity when dropping out of orbit, despite being rapidly slowed down in the atmosphere. The arc each surviving component travels depends in its mass to area ratio. Additionally, the dispersion of debris is strongly dependent on cross winds that can cause a cross-track spread in debris. Typically, the debris field of a re-entering satellite stretches from 500 Kilometers downrange from the Entry Point up to a distance of 1,300 Kilometers, where the most dense components come down.
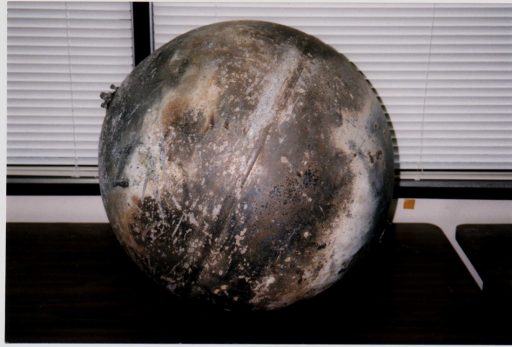
The survivability of specific components depends on a number of factors including the component’s material, shape, area to mass ratio and shielding provided by other spacecraft components. A generalization that is often found states that aluminum components usually burn up completely unless they have a high area to mass ratio, are shielded by other satellite parts or are released late in the entry process. Titanium or stainless steel spheres (tanks) or solid rocket motor casings have a good probability of surviving and hitting the Earth’s surface.
For the Kanopus ST satellite and Volga Upper Stage, with a combined mass in excess of one metric ton, a number of components are likely to survive re-entry – in particular small spherical pressurant and propellant tanks of the upper stage as well as components of the main engine. Also likely to survive are the three reaction wheels of the spacecraft which represent dense metal disks that can easily withstand the re-entry environment. Some bus structure components may also survive.
.
Observing Re-Entry
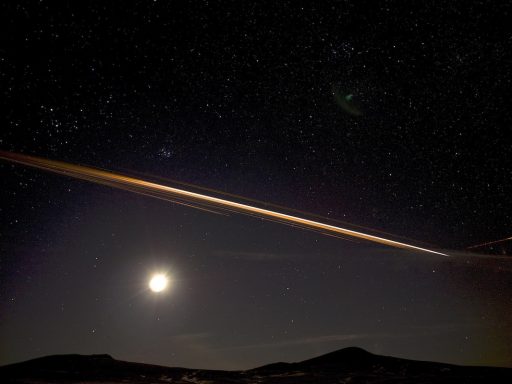
As described above, predicting the exact timing of re-entry is nearly impossible, but the re-entry window that is issued based on orbital tracking can help when trying to watch the event. The exact location of re-entry can not be predicted, however a few hours before the event, zones will be excluded. Should you have Kanopus/Volga passes around the time of final entry predictions that are made on the day of the estimated Re-Entry, you should certainly step outside and look for the spacecraft. Websites like Heavens-Above.com provide a list of passes for any given location on Earth. (Pass Predictions of these websites are based on orbital information that is updated several times per day. On Re-Entry day, the spacecraft’s orbit decays rapidly so that these predictions become inaccurate very fast and the time of the start of the pass might vary by several minutes.) When re-entering, the disintegrating vehicle will streak across the sky with a visible plasma tail and glowing debris falling back to Earth.
How the Orbital Tracker Works
There often are misconceptions on how tracking websites like n2yo.com work. A common believe is that these ‘real time tracking sites’ indeed show the position of the satellite based on tracking information gathered in real time. This is not the case. The trackers receive a set of orbital elements from which the position of the satellite at any given time is calculated. These orbital elements are generally updated several times per day to show the position of the satellite with reasonable accuracy.
However, with a spacecraft close to re-entry, the fast reduction of orbital period will result in the tracker and the actual position of the satellite to get out of synch between tracking updates. Therefore, it is common that the satellite will appear up to a few minutes before the predicted times according to online observation calculators or trackers. Also, when re-entry comes, online trackers will continue to show the propagated position of the satellite for several hours or even days, although the satellite has long ceased to exist.