Max Valier Sat

The Max Valier Sat is a 15-Kilogram NanoSatellite developed in a collaboration between two vocational schools, satellite builder OHB Systems and the Max Planck Institute to conduct a scientific mission with an X-Ray astronomy payload.
The two schools involved in the project are both located in South Tyrol, Gewerbeoberschule “Max Valier” in Bolzano and Gewerbeoberschule “Oskar von Miller” in Merano. OHB Systems provides technical support and secured the launch opportunity for the satellite as a secondary payload on an Indian PSLV rocket. The Max Planck Institute is responsible for the development of the µRosi Miniature X-Ray Telescope which represents the first spaceborne X-Ray sensor to be accessed by the amateur astronomy community.
The small satellite is based on a flat satellite platform developed by OHB in cooperation with the German Aerospace Center, also used by the Venta-1 and AISat-1 missions. This design provides a form factor of 40 by 40 by 10 centimeters.
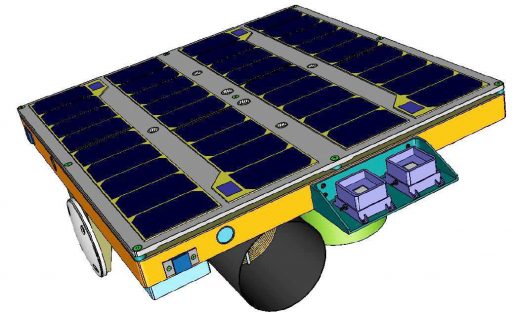
Originally, the satellite was to fly with three payloads – the X-Ray Telescope, a receiver for the Automatic Identification System for ship-tracking and a CMOS camera for Earth-imaging, however, both the AIS payload and camera were dropped during development.
The satellite’s two principal components are a top deck hosting the solar panel, comm antennas & attitude sensors, and a Payload & Support module mounted on the bottom of the larger top deck. Power generation is accomplished with six strings of ten solar cells with a 30% efficiency, generating an average power of 10 Watts with peaks at 45W. Power is delivered to a Lithium Ion Battery and from there distributed to the various satellite subsystems.
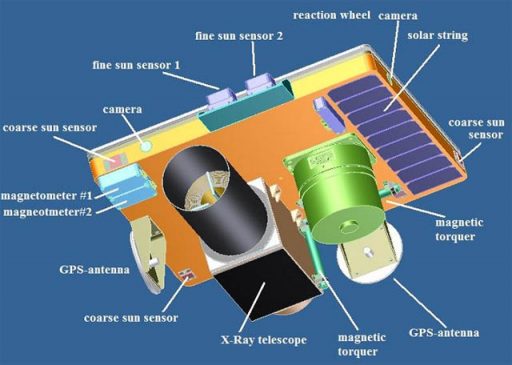
Attitude determination is accomplished with a Coarse Sun Sensor, Fine Solar Aspect Sensor and three-axis magnetometer. The Coarse Sun Sensor is essentially a cube with a solar cell on each face to determine an approximate solar vector by comparing the current coming from each of the cells. The fine sun sensor is the primary attitude sensor, achieving an accuracy of 0.1 degrees.
The magnetometer is responsible for measuring the magnetic field vector and strength to deliver data needed for the actuation of three magnetic torquers that run a current through coils in the presence of Earth’s magnetic field to generate a directed torque.
A single momentum wheel is installed on the satellite’s spin axis (normal to the solar panel) to control the spacecraft’s spin rate since Max Valier typically operates in a spin-stabilized mode with the solar arrays pointed to the sun and the X-Ray sensor sweeping across the sky.
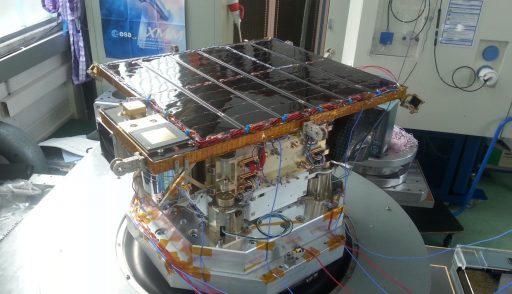
The brain of the Max Valier Sat is a 16-bit Microcontroller in charge of commanding all onboard systems, processing attitude sensor data and collecting data from the payload. The OBC is a proprietary design of GOB Bolzano and offers eight serial interfaces and other ports to communicate with the satellite systems. Communications with the ground are accomplished in the amateur VHF/UHF bands for telemetry downlink and command uplink (145/450 MHz) while data from the payload is downlinked through S-Band at the 2.4GHz frequency and higher data rates.
µRosi is the smallest spaceborne X-Ray Telescope flown to date, weighing in at only 2.4 Kilograms. The primary task of the instrument is to perform an all-sky survey of the observable universe in the soft X-Ray band at an angular resolution of 1°. Despite its small size, µRosi achieves an excellent energy resolution matching that of current flagship X-ray missions like Chandra and XMM-Newton, though the spatial resolution of the small telescope can not reach the performance of large satellite-based X-Ray telescopes.
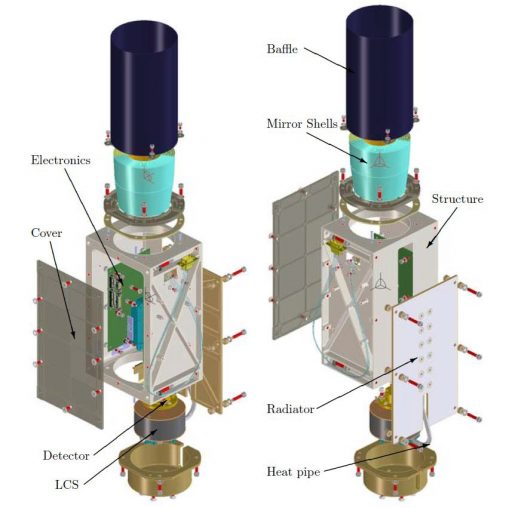
µRosi has an aperture diameter of eight centimeters and comprises a series of components – an entrance baffle, a mirror shell assembly, the detector assembly, an electronics assembly and a thermal regulation system. The optical bench of the telescope is a cross-sectioned structure made of carbon-fiber-reinforced polymer selected for its mechanical stability and thermal insulation. Aluminum interfaces are used for the detector and mirror modules and shimmed to align the optical components.
The µRosi telescope employs a Wolter-I optical design that uses only grazing incidence optics because the conventional telescopes employing lenses or mirrors are of no use for X-rays – transparent lenses have a refractive index substantially different than one whereas X-rays require refractive indices very close to one, and mirrors, when used at near-normal incident angles, will not reflect the x-rays, instead transmitting or absorbing them which is of no use when trying to focus X-rays onto a detector.
Therefore, X-ray optics are only useful if the angle from the plane of reflection is very low, typically below 2 degrees. These grazing incidence mirror arrangements were first outlined by Hans Wolter in the 1950s with the Type I design being the most frequently used option in modern space-based X-ray observatories.
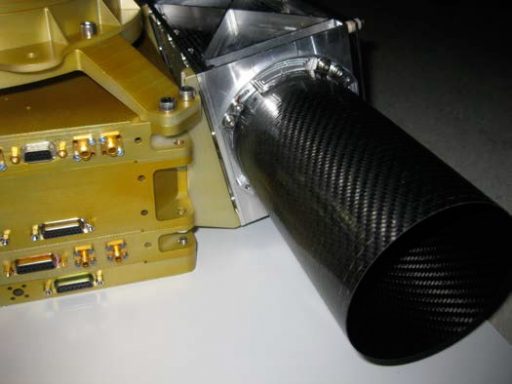
µRosi uses 12 nested double-cone mirror shells made of Gold-coated Nickel by electro-galvanic forming – a process developed for the eROSITA X-Ray telescope. The six outer mirror shells have a thickness of 0.3 millimeters and the inner six are 0.2mm thick in a design trade-off between stability and optical performance. The optical design of µRosi creates a focal length of 245 millimeters, dictated by the available payload envelope on the small satellite.
The µRosi telescope features a non-imaging silicon drift detector, meaning that the detector effectively only has a single pixel and the field of view of the focusing telescope determines the spatial resolution of 1°. This resolution, while not reaching that of operational large-satellite missions, is sufficient for the detection of bright X-Ray sources as well as transient events detected as part of the all-sky survey. It is expected that µRosi will deliver high-resolution energy spectra of around 100 targets in the soft X-ray universe.
The Silicon Drift Detector is a commercial product and has a sensitive area 4.6 millimeters in diameter with an active thickness of 450 micrometers. The detector achieves an energy resolution of 128 electronvolt and operates at a read-out cycle of 1 microsecond. An aluminum coated polyimide filter is placed in front of the detector to reject the visible spectral bands while permitting the desired soft X-rays to pass.
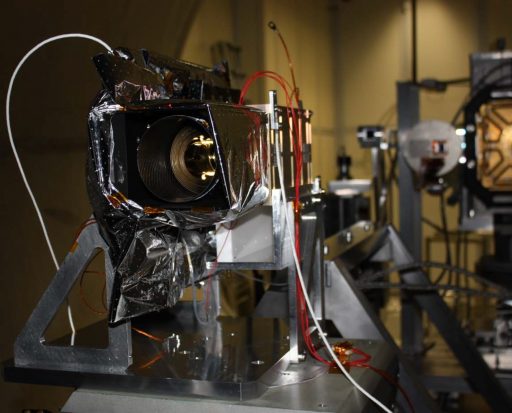
A dedicated electronics board housed within the telescope structure is responsible for the read-out of the detector, processing the signals into digital data and transmitting it to the spacecraft for storage and downlink. The electronics are also in charge of instrument thermal control, power supply and command execution.
Thermal control for the µRosi instrument is relatively complex as the mirror assembly and detector require very different thermal environments. The mirror module is kept at +20°C using heaters to guarantee the precise optical alignment needed to obtain the expected instrument performance. The detector is cooled to -20°C to achieve the optimal spectral resolution, accomplished with a dedicated radiator acting as heat sink and a Latent Cold Storage in use as a buffer to deliver a high temperature stability without relying on a more complex active system. The radiator and buffer are linked with a variable conductance heat pipe while the Latent Cold Storage is hard-mounted to the detector module.
To facilitate the all-sky survey to be performed by the µRosi instrument, Max Valier is flown as a spinning satellite with the telescope sweeping out a great circle across the visible sky with a slow precession of the scan plane to ultimately obtain a full scan of the sky in a period of six months. Because the satellite operates in a low orbit, it will also scan the Earth, allowing it to study the geocorona at energies under 1keV.
For proper context of the X-Ray imagery acquired by the µRosi instrument, Max Valier hosts a Star Camera that is used for proper location-referencing of the telescope images with a precision of 0.06 degrees.
Data collected by the Max Valier Sat will be evaluated and published by amateur astronomers. µRosi can enhance the all-sky catalog provided by the ROSAT mission in the 1990s by adding high-resolution spectral data for bright X-Ray sources; this is possible because of compatible optical design parameters with respect to F-number and energy range between ROSAT and µRosi.