Navigation Augmentation Satellite Ready for Liftoff atop Japanese H-IIA Rocket
The launch of Japan’s H-IIA rocket with the third Quasi-Zenith Satellite had to be scrubbed deep into the countdown on Saturday due to a potential issue with the launcher’s propulsion system. >>Full Update
——————–
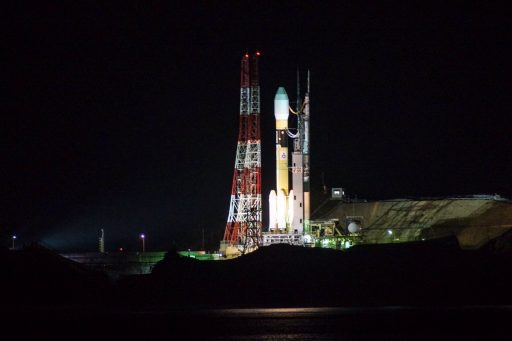
A satellite designed to improve navigation availability and accuracy over Japan is ready for launch on Saturday atop an H-IIA rocket blasting off from the country’s picturesque Tanegashima Space Center. Michibiki-3 is the third satellite to join the Quasi Zenith Satellite System designed to augment the U.S. Global Positioning System and Europe’s Galileo for users in Japan’s urban canyons and improve navigation accuracy throughout the Asia-Pacific Region.
2017 is a big year for the Quasi-Zenith Satellite System (QZSS) with three satellites set to join the initial prototype spacecraft launched in 2010 to establish the fully operational system consisting of three satellites in specialized elliptical orbits with high elevation angles for Japan and a single bird in Geostationary Orbit to provide 24/7 augmentation over a broad region. The deployment of the operational QZS constellation marks the culmination of 15 years of development work and more additions are planned later on to deliver further availability and accuracy enhancements.

Saturday’s launch has an exceptionally long window opening at 4:40 UTC and extending eight and a half hours to send QZS-3 into a Geostationary Transfer Orbit to become the sole GEO satellite of the constellation as a constant navigation beacon over the Asia-Pacific Region. As the heaviest of the QZS satellites, Michibiki-3 rides on an H-IIA 204 rocket that enlists the help of four solid rocket boosters to send the 4,700-Kilogram craft on its way. H-IIA will be in action for 28 minutes and 40 seconds to dispatch QZS-3 into its highly elliptical target orbit via two burns of the rocket’s upper stage.
Development of the Quasi Zenith Satellite System started back in 2002 with the overall objective of increasing the availability of GPS navigation services, particularly in the country’s urban canyons where tall buildings block all GPS satellites that are not at high elevation, potentially causing interruptions in service. To that end, QZSS proposed to establish a three-satellite constellation in a specialized orbit that places one satellite directly over Japan at any given time.

The QZSS satellites are designed to deliver two types of signals – GPS-compatible navigation signals and GPS augmentation messages that can be used by ground receivers to more accurately calculate user position. Through the GPS-compatible messages in the L1, L2 and L5 bands, the QZSS satellite can be treated as an additional member of the GPS constellation at high elevation angle from all locations in Japan, increasing availability of GPS.
The GPS augmentation approach followed by QZSS is similar to that of the U.S. Wide Area Augmentation System in that a number of ground stations monitor variability in GPS signals – caused by clock errors and ionospheric delay – and provide correction messages to a high-altitude satellite that immediately relays them to augmentation-enabled receivers on the ground to increase location accuracy to around one meter.
The first QZS satellite served as a pathfinder and underwent extensive signal characterization and fine tuning before being moved into active service nine months after launch. Studies showed that GPS accuracy was improved by a factor of 2.5 when QZS-1 was overhead and transmitting augmentation code. These results prompted JAXA to order three DS-2000-based satellites from manufacturer Mitsubishi Electric in 2013 for a total of $526 million to establish the QZSS constellation for 24/7 augmentation services, also expanding from an initial three-satellite design to a four-satellite constellation with one craft in Geostationary Orbit to broaden the accuracy augmentation zone to the entire Asia-Pacific Region.
>>QZSS Satellite and Constellation Overview
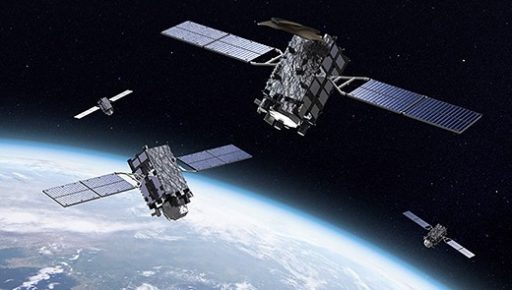
QZS-3 as the geostationary member of the constellation weighs 4,700 Kilograms and measures 5.4 x 3.2 x 4.1 meters in size when in its stowed configuration and 19 meters from tip to tip when extending its two power-generating solar arrays. At the heart of the satellite sits a pair of redundant Rubidium Atomic Clocks that provide the ultra-stable timing needed in the generation of regular GPS navigation messages. Japan received clearance from the U.S. in 2006 to use the GPS navigation bands as well as the L1-SAIF augmentation signal architecture.
QZSS takes a unique spot in the satellite spectrum in that it is designed to augment another satellite constellation but moves beyond acting as a bent-pipe relay of ground-generated augmentation signals and in-fact delivers navigation signals of its own. The QZSS constellation will also be capable of augmentation for the European Galileo navigation constellation that will become operational before the end of the decade. QZSS itself will be fully operational by mid-2018 and JAXA plans to add another three satellites in the 2020s to further increase GPS availability in Japan’s urban canyons.
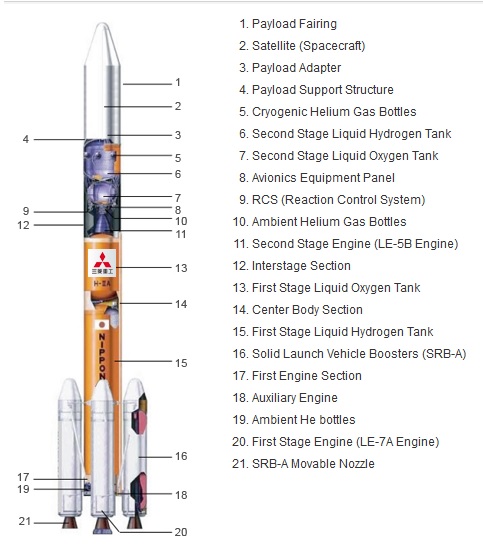
The launch of the QZS-3 satellite will be the fourth flight of the H-II rocket family in 2017, having already lifted the DSN-2 defence communications satellite, the IGS Radar 5 reconnaissance satellite and the QZS-2 craft. It will be the second flight of the more-powerful 204 variant in 2017 and the 35th launch of the H-IIA since its introduction in 2001.
H-IIA 204 consists of a two-stage stack measuring four meters in diameter plus four SRB-A boosters clustered around the first stage. The vehicle stands 53 meters tall, weighs 443 metric tons at liftoff and lifts up to 15 metric tons into Low Earth Orbit and six tons into Geostationary Transfer Orbit.
>>H-IIA Launch Vehicle Overview
H-IIA emerged from its assembly building at the midnight hour for a 30-minute trip to cover 500 meters to Launch Pad 1 at the sea-side launch complex. Once at the pad, the rocket’s Mobile Launch Platform will be hooked up to propellant, power and data interfaces and undergo power-up operations for an initial series of checkouts. H-IIA will press into propellant loading around seven hours and 45 minutes before the opening of the day’s launch window, pumping 120 metric tons of -183°C Liquid Oxygen and -253°C Liquid Hydrogen into the two cryogenic rocket stages.
After the three-hour fueling sequence, H-IIA will be kept topped up at flight level while engineering teams work through a comprehensive list of checkouts to clear the vehicle for a 60-minute terminal countdown operation that will transition to a fully computer-controlled mode at X-4.5 minutes to put the vehicle through tank pressurization, transfer to internal power and the control handoff from the ground to the rocket’s computers.
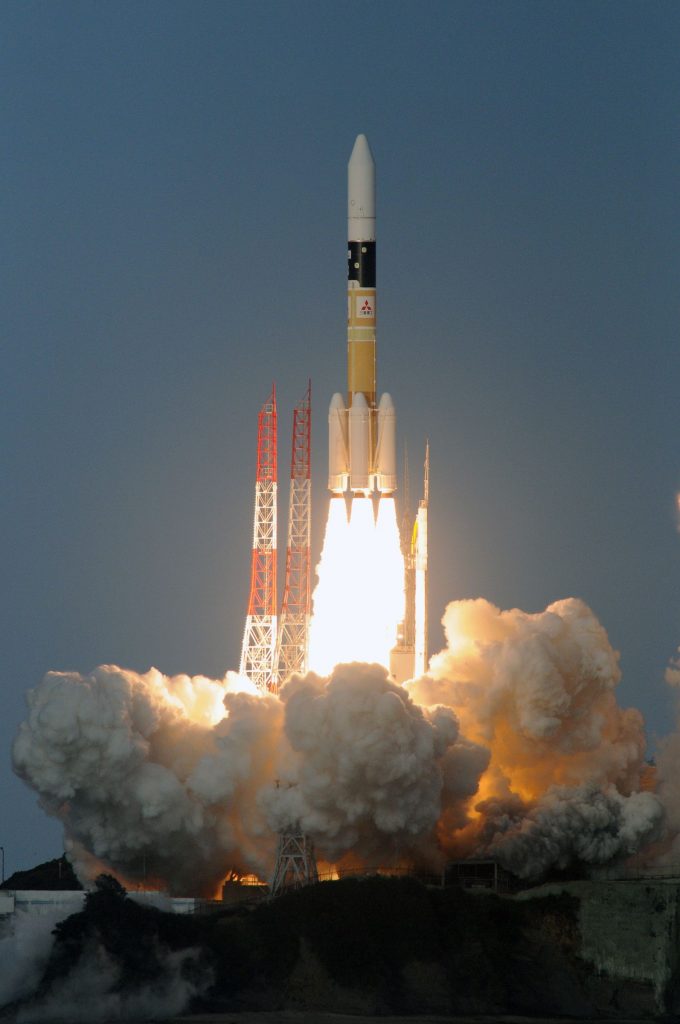
H-IIA will start breathing fire at X-5.2 seconds when the LE-7A main engine will soar to life, spinning up its turbopumps and building up thrust under close supervision of flight computers to ensure the engine reaches operational conditions before the boosters are ignited – committing the vehicle to flight.
At the moment of booster ignition, H-IIA will shoot off the ground with a total thrust of over 1,030 metric ton force – the boosters delivering almost 90% of the rocket’s thrust at blastoff. With an initial thrust to weight ratio higher than two, H-IIA will climb vertically for only a handful seconds before beginning to pitch and roll in order to get aligned with its precisely planned ascent path taking the launch vehicle to the south-east for a trip across the Pacific Ocean, heading to a Low Earth Parking orbit.
The four boosters will fire for 108 seconds, in the process burning 66 metric tons of solid propellants and helping H-IIA accelerate to 2.2 Kilometers per second. Burnout will be sensed by the declining pressure in the combustion cambers and separation will be initiated two minutes and six seconds into the flight for the first two boosters and three seconds later for the other two, using the booster thrust struts to ensure a clean separation of the 15.1-meter long SRBs.
Powered flight continues on the LE-7A engine of the first stage, generating a thrust of 109 metric ton-force when flying through the rarefied upper atmosphere, consuming 260 Kilograms of cryogenics each second of powered flight. Three minutes and 45 seconds into the flight, H-IIA will be over 170 Kilometers in altitude and split open its five-meter payload fairing as aerodynamic forces can no longer harm the delicate spacecraft structure.

The first stage will finish its job six minutes and 38 seconds into the flight, having boosted the vehicle’s speed to 5.9 Kilometers per second. Eight seconds after cutoff, the 37-meter long first stage will drop away to clear the way for ignition of the second stage’s LE-5B engine at X+6:52, soaring to a vacuum thrust of 13,970 Kilogram-force to lift the stack into a lightly elliptical Parking Orbit via a burn of four minutes and 31 seconds.
After completion of the first propulsive phase of the mission, H-IIA will enter a coast phase of approximately 12 minutes to continue on its south-easterly path in order to fire the engine again when passing the equator so that the high-point of the orbit can be placed above the equator as well – standard practice in GTO launches. The second burn is targeting ignition 23 minutes and 39 seconds after launch when H-IIA will be 370 Kilometers in altitude.
The second burn of the 11-meter long second stage has a planned duration of four minutes and 10 seconds to raise the vehicle’s velocity by 2.5 Kilometers per second and significantly lift the high point of the orbit. H-IIA F35 is aiming for an orbit of 380 by 35,976 Kilometers at an inclination of 20 degrees with spacecraft separation expected 28 minutes and 40 seconds after liftoff.
The spring-loaded separation of the satellite will mark the start of its first steps in orbit – going through a stabilization maneuver and initiating communications with the ground for initial health checks that will clear the way for a multi-burn climb into Geostationary Orbit.