ASIM – Atmosphere-Space Interactions Monitor

ASIM, the Atmosphere-Space Interactions Monitor, is an ESA science instrument taking up residence outside the Columbus Module of the International Space Station to study Transient Luminous Events (TLEs) in Earth’s upper atmosphere like Blue Jets, Red Sprites and Elves via a suite of cameras and photometers sensitive in a broad wavelength range to reveal previously unknown details of the electrical and chemical processes ongoing where the atmosphere and space interact.
The first proposal for the ASIM concept came from the Danish National Space Center (DNSC) back in 2007 and, since then, the project has been worked on by Danish, Norwegian and Spanish institutions to advance its design. ESA eventually provided approval for the instrument to be flown as an ISS external payload, operating from an Earth-pointed position on the Columbus External Payload Platform.
Transient Luminous Events were first observed in 1989, occurring in the Stratosphere and Mesosphere as companions of thunderstorms in the Troposphere.

These events are of particularly short duration, hence their name, and can be broken down into blue jets, sprites and elves by their appearance and the altitude at which they occur. Researching these phenomena from space has been a difficult endeavor given the relatively low frequency and spatial distribution, though ISS is considered a perfect platform due to its non-synchronous orbit, allowing locations to be observed at different times of day.
In recent years, the first proper still images and videos of sprites and blue jets were collected by astronauts aboard the International Space Station and there have been attempts to study these phenomena via small satellite missions (e.g. the Firefly CubeSat and Japan’s Rising 2) or through observation posts set up on mountains above thunderstorm clouds. In 2012, a bright red sprite was captured over a thunderstorm above Myanmar and in 2015, the Thor experiment, operated by ESA Astronaut Andreas Mogensen, captured the first-ever high-resolution video of a pronounced blue jet – illustrating how little is actually known about these phenomena.

ASIM combines two cameras, three photometers, an X-ray detector and a gamma-ray instrument to study the atmosphere as one system from the surface to the edge of space to help understand the coupling between phenomena in the dense atmospheric layers and responses in the upper atmosphere.
ASIM is looking back at a fairly lengthy road to launch, starting development in 2007 with involvement of DNSC and the universities of Valencia, Spain and Bergen, Norway. The instrument moved through Phase B of its design process in 2009 and entered the production phase in 2010 after Critical Design Reviews were passed. Initially working toward a launch in 2014, ASIM suffered a combination of delays – most notably a complete re-integration of the structure after the failure of the Columbus External Payload Adapter (CEPA) that had to be replaced in full.
The primary objectives of ASIM are several-fold: 1) study the physics of TLEs through optical detection with high spatial and temporal resolution in selected spectral bands; 2) study Terrestrial Gamma-ray Flashes (TGFs) and their connection to TLEs and thunderstorms via X- and gamma-ray detection with high temporal resolution and a broad energy range down to 10 keV; 3) simultaneous detection of thunderstorms, TLE and TGF activity to enable a study of the coupling to the mesosphere, thermosphere and ionosphere, and 4) collect at least one full year of observation data across all local times to allow seasonal and local time variations to be assessed.
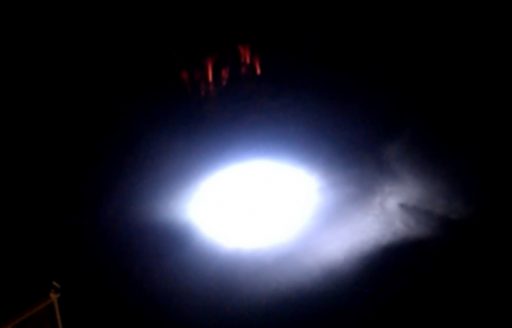
In addition to its main objectives, ASIM is hoped to address secondary objectives like the spectroscopic study of the aurora, measurements of greenhouse gas concentrations above thunderstorms (particularly ozone and oxides of nitrogen), and detecting the optical and spectral signatures of meteors to reveal their chemical composition and origin.
The combination of optical instruments, photometers and specialized instruments for X- and gamma-rays allows ASIM to collect a wide range of measurements: auroral displays will be studied with the optical and X-ray instruments, differential absorption of lightning-illuminated thunderstorm clouds will allow a measurement of ozone column density, NOx generation in TLEs can also be measured photometrically, and a combination of optical imaging and photometry will be used to detect meteors.

The integrated ASIM payload weighs 314 Kilograms and comprises the CEPA (Columbus External Payload Adapter) as its central structural element, three modules making up the MMIA (Miniature Multispectral Imaging Array) and the single MXGS module (Miniature X-ray and Gamma-ray Sensor). Two of the MMIA modules are pointed to the ram direction (forward) while one is nadir-oriented (Earth-facing) to match the boresight of MXGS.
The CEPA provides structural attachment to the Columbus External Payload Platform through its FRAM (Flight Releasable Attachment Mechanism) which also forms the electrical and data bridge between ISS and the ASIM payload. ASIM receives raw ISS power at 120 Volts, routed to the Data Handling and Power Unit (DHPU) that builds the central element of the instrument payload, tasked on the one hand with converting the operational ISS power supply to the 28-Volt instrument power bus and on the other hand with handling all instrument data – processing commands from the ground relayed via the ISS data system and delivering housekeeping telemetry and pre-processed image data from the various detectors of the ASIM instrument. Data interfaces used by ASIM include a 1553-MIL-STD bus for housekeeping data and time synchronization, an Ethernet connection for science data and a serial line that allows for updating the DHPU firmware by the ISS crew.
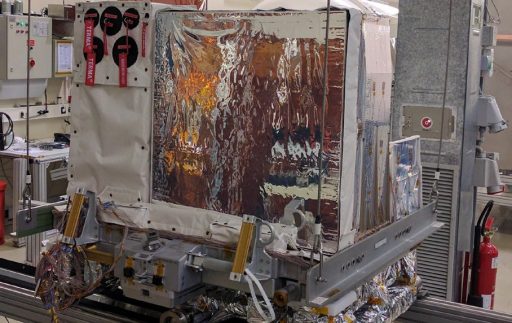
ASIM has a nominal power consumption of 200 Watts during science operations plus an additional 230 Watts needed for the survival heaters; its downlink data allocation is a continuous stream of 200 kbit/s which will be fully utilized and the instrument also employs onboard data prioritization since the link will not be sufficient to deliver all collected data to the ground.
The scientific payload carried by ASIM is made up of the three MMIA modules and the single MXGS module – all in all comprising six optical cameras, six photometers, one X-ray and one gamma-ray instrument. MMIA has two groups of optical instruments pointed to the limb/ram direction and directly towards nadir, operating in carefully selected bands to filter out data with Transient Luminous Events and reject ordinary lightning events through the use of a sophisticated onboard algorithm.

Two MMIA units, each with two optical imagers and two photometers, point toward the ISS direction of motion to view Earth’s limb while one unit points nadir (directly to Earth), co-aligned with the MXGS instrument. The cameras are all identical with the exception of their spectral bands and bandwidths which have been uniquely selected for each camera; the same is the case for the photometers.
The Cameras have baffles for straylight rejection and employ 1024 x 1024-pixel frame-type Charged Coupled Device detectors. All MMIA cameras collect 12 frames per second across a 20 x 20-degree field of view for the limb cameras and 80 x 80° for the wide-field cameras pointing nadir, translating to a ground resolution of 300-600 meters for the limb cameras and 300-400 meters for the nadir cameras.
The four limb cameras employ three narrow-band channels at 336.2, 391.4 and 762.2 nanometers with a bandpass of 5.0 nm and one wide-band channel sensitive from 650 to 740 nanometers. The nadir-viewing cameras operate at 337.0 and 777.4 nanometers with a bandwidth of 5 nm.
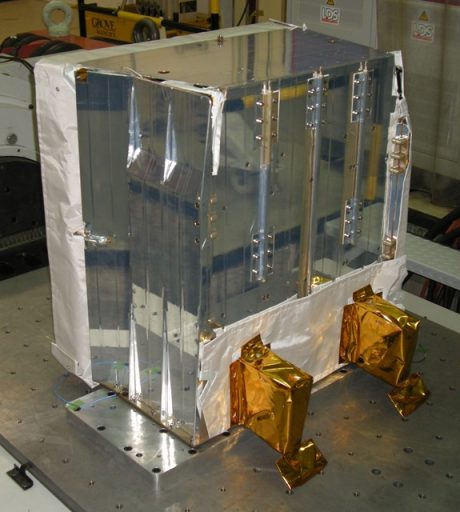
The MMIA photometers are tasked with the measurement of rapid time variations which is not possible with the cameras. They view the exact same region as the cameras but measure only the total photon flux across their field of view at a frequency of 100 Hz. The limb-pointing photometers operate narrow-band channels at 236.6, 337.0 and 391.4 nanometers plus the same wide-band channel as the cameras at 650-740 nm while the nadir-viewing photometers comprise a narrow-band unit sensitive at 337.0 +/-5 nm and a broadband UV photometer at 145-250 nanometers.
MXGS is in a nadir-viewing position to look directly down upon terrestrial thunderstorms to detect radiation from Terrestrial Gamma Flashes (TGFs) and lightning-induced electron precipitation. The instrument has an active area of 32 by 32 centimeters and employs BiGe (Bismuth Germanate) scintillator and CZT (Cadmium-Zinc-Telluride) semiconductor detectors to collect spectral data. One critical requirement for MXGS was a fast electronic circuitry since the events to be detected and recorded only last for one to five milliseconds and additional provisions are needed to have an event synchronization trigger between the nadir-pointed MMIA module and MXGS.
MXGS is only available in a nadir-pointed configuration for the simple fact that a limb-viewing version would not be effective given the atmosphere’s attenuating properties for X- and gamma-rays. Pointing nadir, MXGS has a minimum of atmosphere between the detector and the source within its field of view.
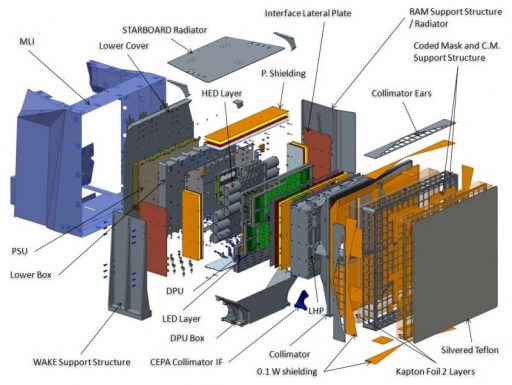
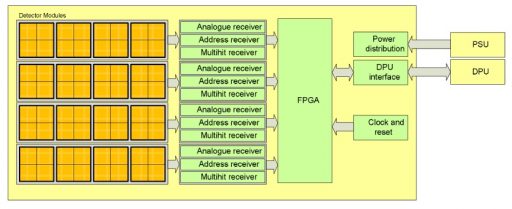
The MXGS instrument has a low-energy detector sensitive from 15 to 400 kilo-electronvolt (CZT) and a high-energy detector covering energies of 200 keV to 40 MeV (BGO). The low-energy detector is pixelated through a 128 x 128 array and has a high-density coded mask placed in front of it so that post-processing algorithms can locate the TGF source with sufficient accuracy for correlating with MMIA imagery.
The MXGS detector consists of a 1024 cm² CZT crystal array that is protected against the background by a passive shield surrounding the detector housing on all sides except the optical axis. The 80 x 80° field of view is defined by an 11-centimeter hopper-shaped collimator assembly.
Placed right underneath the detector plane is the Electronics Box containing the Detector Front End Electronics (DFEE) comprising four Detector Assembly Units (DAUs), each in turn facilitating 16 Detector Modules (DMs) and one Detector Assembly Board.
The Detector Module hosts 256 CZT pixels (16 x 16, 2.5 mm pitch) tiled together on Arlon substrate and connected to an Application-Specific Integrated Circuit tasked with the fast read-out needed for the detection of the short-lived events of interest. The DAU arranges the 16 DMs to create a 64 x 64-array, all connected to the Detector Assembly Board where the events are read-out and transferred to the DPU.
Underneath the 4096 x 4096-pixel, 0.5 mm thick CZT layer sits the high-energy BGO scintillator detector, 900 cm² in area and 3.2 centimeters thick to support detection energies up to 40 MeV. BGO converts particle energy to photons at visible wavelengths and photomultiplier tubes coupled to the BGO bars on each end detect the light impulses which are converted to electrical signals that can be digitized and recorded.

The BGO detector layer comprises four BGO Detector Assembly Units, each consisting of three BGO crystals with their corresponding photomultiplier tubes (PMTs) and read-out electronics. The PMTs are connected to preamplifiers to multiply the electrical signal and transmit it to the analog-to-digital conversion stage from where the digitized data is sent to a Field Programmable Gate Array, one in each BGO Detector Assembly Unit.
The FPGA performs offset subtraction and peak detection to identify pulses of interest which are then processed into a data packet with a 1 µs resolution time-tag and delivered to the Data Processing Unit through an 18 Mbps serial link.