Interplanetary Transport System – Spaceship & Tanker

SpaceX’s Interplanetary Transport System (ITS) aims to establish an operational transport capability for crews and cargo to and from Mars to support the planet’s exploration and lay the foundation for a self-sustained civilization on our neighbor in the Solar System.
The ITS concept comprises three principal components – a reusable Booster, an Interplanetary Spaceship and a Tanker. Per the concept’s design, the Spaceship will be launched atop the 77.5-meter tall booster and act as a second stage, in the process expending its propellant supply to reach a Low Earth Orbit. Lingering in orbit for a period of days, the Spaceship is visited by up to five Tanker missions to have its propellant tanks re-filled to make its way to Mars.
While the Booster and Tanker only operate in near-Earth space, the Spaceship will have to support crews of 100+ for a trip to Mars that, on average, lasts 115 days and culminates in a daring landing maneuver employing a combination of aerobraking in the tenuous Martian atmosphere followed by a propulsive landing on the surface.
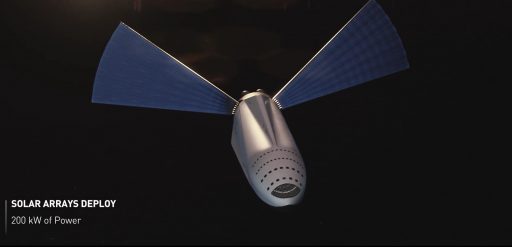
Over the course of a ~20-month surface mission, the Spaceship has to be refueled with propellants generated from resources on Mars to accomplish the return trip to Earth by blasting off from Mars, achieving a trans-Earth trajectory, re-entering Earth’s atmosphere and again landing propulsively atop three deployable landing legs.
As evident in the baseline mission design, the Spaceship has to be a real multi talent – fulfilling a series of functions: second stage to orbit, interplanetary spacecraft, Mars entry and propulsive landing, surface habitat, Mars ascent vehicle, Earth re-entry and landing.
Interplanetary Spaceship
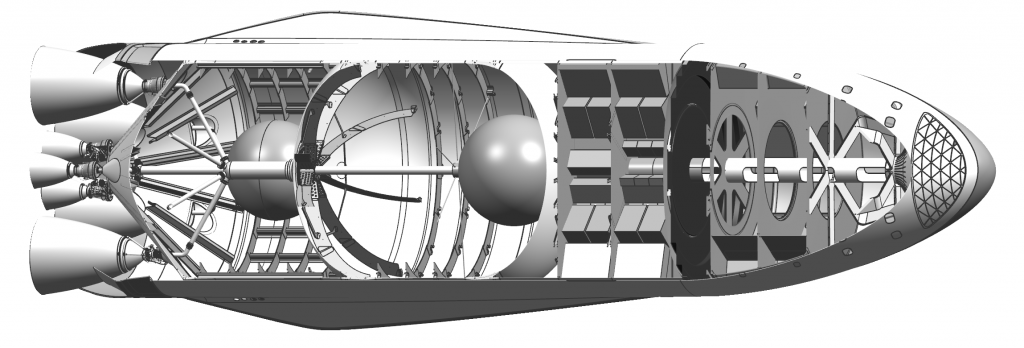
Type | ITS SpaceShip |
Length | 49.5 m |
Diameter | 17 m |
Dry Mass | 150 t |
Propellant Mass | 1,950 t |
Cargo & Crew Mass | 300 t |
Total Launch Mass | 2,400 t |
Oxidizer | Sub-Cooled Liquid Oxygen |
Fuel | Sub-Cooled Liquid Methane |
Oxidizer Mass | 1,535 t |
Fuel Mass | 415 t |
LOX Temp (Est.) | 66 K |
LCH4 Temp (Est.) | 93 K |
Propellant Fraction | 0.81 |
Propulsion | 6 Raptor Vac + 3 Raptor SL |
Raptor Vac Thrust | 3,500kN |
Raptor SL Thrust in Vac | 3,295 kN |
Raptor Vac Impulse | 382 Sec |
Raptor SL Impulse in Vac | 361 Sec |
Total Thrust | 31 MN |
Attitude Control (Burn) | Gimbaled Raptor SL Engines |
Attitude Control (Vac Burn) | Differential Throttling of Vac Engines |
Attitude Control (Cruise) | Reaction Control System (O2 & CH4) |
Burn Time | >322 Seconds |
Burn Time (SL Engines) | >54 Seconds |
Total dV (Est.) | 6.5km/s (Fully Loaded) |
The ITS Spaceship stands 49.5 meters tall and across its length is split about 45/55 between usable crew/cargo volume and the propulsion compartment. It most likely shares the first-stage’s tank diameter of 12 meters but measures 17 meters wide, employing an aerodynamic lifting body for Mars and Earth Entry.
The Spaceship has a launch mass of 2,400 metric tons, including a dry mass of 150 metric tons and 300 tons of crew support and cargo.
The ITS Spaceship – in a later design iteration – offers room, resources and life support capability for crews of over 100 humans. In Elon Musk’s 2016 presentation revealing the SpaceX Mars Architecture, the primary focus was on the aspects of transporting humans to and from Mars and items such as crew operations and life support for the lengthy flight through deep space were only briefly discussed.
The Spaceship’s design calls for a large panoramic window on the upper forward-facing side of the vehicle, plus a large number of smaller windows, possibly one for each crew quarter. The Crew Compartment is divided into a large cargo hold in the aft and the actual crew area in the forward section of the spacecraft. A tube runs the length of the crew compartment as a central structural element that, from its appearance, also functions as a tunnel for crew transfer between different levels within the cabin.
The ITS Spaceship hosts a sophisticated propulsion system with a tank-in-tank feature and a combination of sea-level and vacuum-optimized engines.
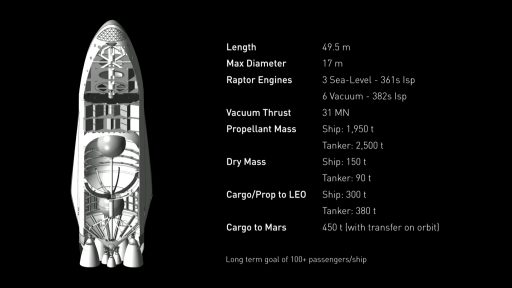
SpaceX’s design mantra since the early days of the company – ‘simplification through commonality’ – also shows up in the design of the Spaceship. The very similar tank design on the Booster, Spaceship and Tanker allows the same tools and manufacturing techniques to be used, streamlining the production process and reducing development cost. The Raptor engine is common across all three ITS components and is likely to become the first engine to be in operational use in Earth’s atmosphere, space, and the Martian atmosphere.
Six methane-fueled Raptor Vac engines with large nozzle extensions are installed on an outer ring that surrounds a cluster of three sea-level engines, creating a propulsion system that is efficient for interplanetary maneuvering in deep space as well as landing in atmospheres of different composition.

Two large composite propellant tanks reside in the aft compartment of the Spaceship, employing the common design with the oxidizer tank installed atop the fuel tank. Both tanks are using composite structures with ring frame supports on the inside.
In the initial reveal of ITS on September 27, 2016, Elon Musk presented a ground test unit of the Spaceship’s Liquid Oxygen tank which will ultimately sit atop the vehicle’s Methane tank. The oxidizer tank features spherical bulkheads, the lower of which serves as a common bulkhead between the two tanks in a mass-saving measure. The fuel tank consists of a cylindrical and conical section that permits mounting of the taller Raptor Vac engines on the perimeter of the vehicle and ensure the nozzle planes of the three smaller Raptor SL engines is flush with the Vac engines.
The most interesting item seen in the first technical image released of the Spaceship is a tank-in-tank feature. Both tanks, LOX and Methane, host rather large spherical tanks over five meters in diameter, installed in the upper tank sections. Running aft from the larger LOX Tank Sphere is a feedline which appears to be routed separately from the main LOX feedline and only interfaces with the central three Raptor engines while the six Raptor Vac engines receive propellant from the main LOX tank.
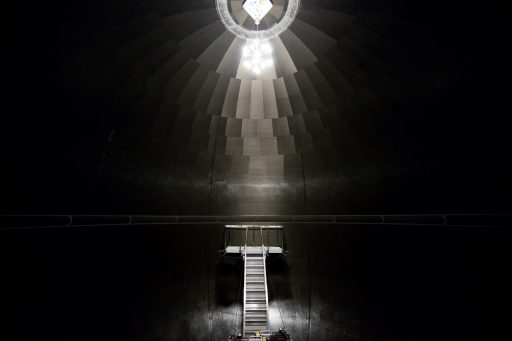
The volume of the spheres is in excellent agreement with a ~3.7 oxidizer-to-fuel ratio employed by the Raptor engines, adding further evidence that these are indeed intended to hold propellant for consumption by the three Raptor SL engines during the Mars and Earth landing maneuvers.
According to technical documentation, the Spaceship’s tanks can hold 1,950 metric tons of Liquid Oxygen and Liquid Methane propellants. The Spaceship, like the ITS Booster, makes use of sub-cooled propellants with the motivation of densifying the liquid propellants to fit a greater propellant mass into the finite tank volume. Calculations presented on this page work with estimated propellant temperatures of -207°C for LOX (same as for Falcon 9) and -180°C for LCH4 (yielding a densification rate similar to LOX, but SpaceX may actually decide to employ slush methane at even higher density, see Prop Section on Booster Page)
The two spheres are capable of holding roughly 150 metric tons of propellants which can power the three SL Raptors for 54 seconds when running at full power.

Based on the design parameters presented for ITS in its initial form, the three SL Raptors will be used during ascent, Mars landing, (possibly Mars ascent) and Earth landing while the Vac engines are employed for ascent, Trans-Martian Insertion, Mars ascent and Trans-Earth Insertion.
Attitude control during cruise flight is provided by a Reaction Control System comprised of a dozen thruster pods and around 40 individual thrusters, consuming oxygen & methane propellants.
In its realistic mission animation, SpaceX shows the ITS Spaceship deploying a pair of fan-like solar arrays capable of generating 200 Kilowatts of electrical power when flying at one Astronomical Unit.
Because the vehicle lands upright on Mars, a crane-like system is needed to deliver crew members and cargo to the surface. According to Elon Musk, SpaceX plans to use a three-cable elevator, taking advantage of the lower gravity on Mars.
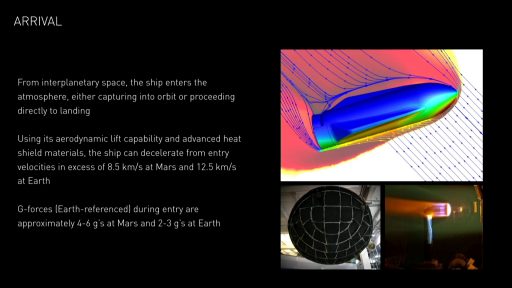
The ITS Spaceship uses a 17-meter wide lifting body to maximize its flight time through the atmosphere at Mars Entry to slow down and minimize the amount of propellant needed for the landing burn. A reusable heat shield based on SpaceX’s PICA-X material will be used. PICA – the phenolic impregnated carbon ablator heat shield – is originally based on NASA technology that SpaceX advanced to create a highly-reusable heat shield that can tolerate the highest entry velocities.
At Mars Entry Interface, ITS is expected to travel over 8.5 Kilometers per second and would use its aerodynamic lift capability to decelerate at a peak Earth-referenced G-force of 6 Gs with peak heating at 1,700°C. Coming back to Earth, ITS would re-enter at a speed of 12.5km/s or more, though through its lifting body design and Earth’s dense atmosphere a more benign entry profile with peaks at 3Gs can be created.
Mission Profile by the Numbers
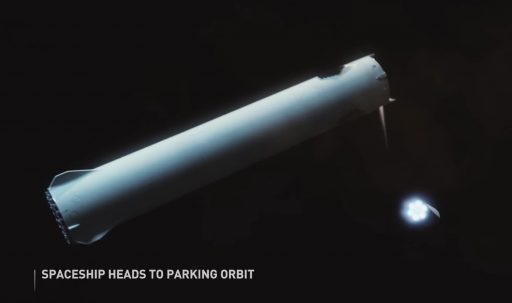
For its launch to Low Earth Orbit, the Spaceship is accelerated to a speed of 2.4km/s by the Booster which also lifts the vehicle outside the dense atmosphere. For the LEO Insertion, the Spaceship initially fires up all nine engines creating a thrust-to-weight ratio of 1.34. It is not possible for the vehicle to reach orbit using the six Vac engines alone taking into account drag and gravity losses, requiring the initial boost of ~54 seconds using the three SL engines to deliver supplementary thrust and burn off the propellant stored in the spherical tanks.
Once in orbit, the Spaceship is visited by five Tanker missions each carrying 380 metric tons of propellant to the vehicle for a total re-fill of 1,900 t, ideally filling the LOX and LCH4 spheres to their full capacity and the main tanks to over 97%.
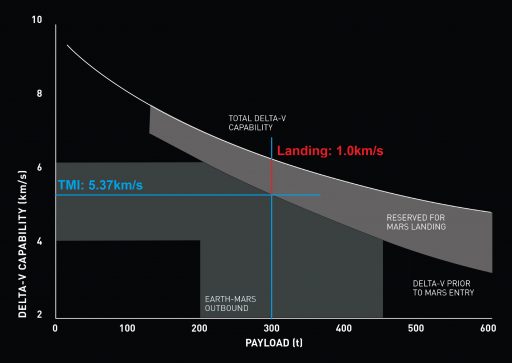
The Trans-Martian Injection utilizes the six Raptor Vac engines only. Per Elon Musk’s IAC 2016 presentation, ITS would use a 6km/s TMI delta-v, however this is likely for an evolved version of the ITS design. The delta-v breakdown shown by Musk points to a 5.37km/s TMI maneuver for the baseline Spaceship design, however, running the numbers for this vehicle version with a 1,900 t re-fill in orbit yields a TMI dV of only 5.1km/s.
Nevertheless, both numbers are above the minimum TMI delta-v requirement of 4.7km/s, though will not permit the Spaceship to conduct an orbit insertion maneuver at Mars though conventional propulsion. Feasible scenarios are a direct atmospheric intercept for landing or capture in orbit using aerobraking in the Martian atmosphere which would add further complexity to the mission.
Also, the lower TMI delta-v will not enable the target travel time of 80 to 115 days, requiring ITS to cruise closer to five months on average.
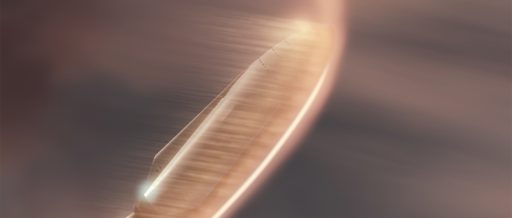
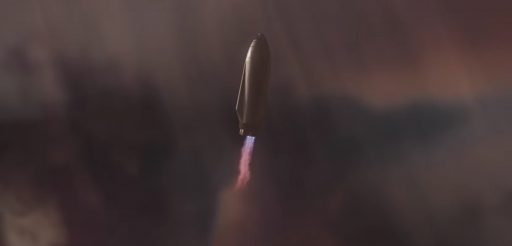
Arriving at Mars, the Spaceship will ideally be holding 150 metric tons of propellant if boil-off from the two spherical tanks can be prevented over the course of the multi-month cruise.
The tank-in-tank design is likely the result of a motivation in preventing boil-off and preserving the propellants at their original temperature.
Assuming the main tanks are fully emptied during the TMI maneuver, they could be re-purposed as thermal barriers for the two spheres. This could be accomplished by venting the tanks down after the insertion maneuver and, in essence, creating a giant vacuum flask in which the propellants are protected from direct thermal radiation and convection while thermal conduction is kept to a minimum.
Employed for the landing maneuver on Mars, the three Raptor engines fed from the spherical tanks can deliver a delta-v of approximately 1.02km/s – a number also shown by Elon Musk during the technical presentation of the 150t Spaceship with 300t of cargo/crew to the surface of Mars.
Over the course of a 20-month surface mission, sufficient quantities of LOX and LCH4 have to be produced and filled into the Spaceship to enable it to depart Mars, boost itself back to Earth and complete a propulsive landing.
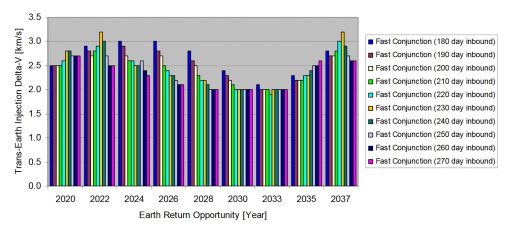
The return from the surface of Mars to a propulsive landing on Earth was completely omitted in Musk’s IAC 2016 address likely because it (while still doable) further pushes the limits.
To make it back to Earth, one first has to get off Mars – either using a Low Mars Orbit Insertion followed by a departure maneuver or a direct departure, both amounting to the same delta-v.
The delta-v required to reach Mars Orbit depends on the architecture of the spacecraft due to specific gravity and drag losses. Numbers for LMO insertion given in literature vary from 3.8 to 4.4 km/s.

Next is the Trans-Earth injection which has a variable delta-v driven by the Earth-Mars geometry for each window as well as the desired travel time. Studies for the 2020-2040 mission windows find a minimum TEI delta-v of 2.0km/s for a 260-day transit in the windows from 2028 through 2033. Faster transits of 200 days and less require a delta-v north of 2.5km/s.
Finally, the Spaceship has to land on Earth in a propulsive maneuver that will require propellants to be preserved through the return journey. Assuming similar landing accuracy and entry guidance precision on ITS and SpaceX’s Dragon would mean that on the order of 44 tons of fuel will be needed for the maneuver.
Working out the numbers for the return trip very quickly shows that additional measures will have to be taken on Mars in addition to filling the tanks up: the Spaceship needs to lose mass – a lot of it.
In a best case return scenario with a dV of 5.8km/s, the ship would need to lose 80 metric tons and for a more realistic return dV of 6.5km/s that number would grow to ~150 tons.
Musk stated, that for each crew member, about one metric ton will be needed in resources and support systems on board the ship and a mass reduction of 150t would still allow ITS to carry 150 t of hardware in addition to the ship’s dry mass and landing propellant. Left behind on Mars would then be trash from the outbound trip & stay plus cargo pre-staged for a future mission. (Mark Watney-inspired note: A 145-day trip to Mars plus 480-day stay would generate approximately eight metric tons of human waste, already a tenth of the minimum mass reduction needed on the spacecraft)
ITS Tanker

The Tanker is a critical part of SpaceX’s Interplanetary Transport System – nobody will go to Mars without picking up a re-fill of propellant near Earth.
Per the ITS Booster and Spaceship design, the ship arrives in orbit with empty tanks, having used almost all of its 1,950-metric ton propellant supply to achieve a Low Earth Orbit. The Tanker is designed for 100 uses, flying back and forth between Earth and LEO to deliver propellant to the Spaceship prior to its departure for Mars. A total of five Tanker trips will be needed for each Spaceship.
The Tanker uses the same design as the Spaceship and launches on the same Booster with the critical change of eliminating the crew cabin and filling the entire interior with tank volume. Because the Tanker missions will be of relatively short duration, no power-generating solar arrays will be needed.
The LOX / Methane propellant combination was in part selected because it permits in-space propellant transfer, however, the transfer of liquids at the quantities needed by ITS will require an extensive development effort including orbital demonstrations.

The ITS Tanker shares the Spaceship’s overall dimensions, standing 49.5 meters tall with a maximum diameter of 17 meters, also employing a combination of six Raptor Vacuum and three Sea Level Engines. Because the interior of the Tanker only consists of composite propellant tanks, its dry mass can be reduced to 90 metric tons.
2,500 metric tons of propellant are carried by the tanker for use during its own mission plus 380 t of propellant upmass that can be transferred to the Spaceship.
When the transfer of propellant is complete, the Tanker conducts a deorbit burn using three of its Raptor Vac engines followed by a propulsive landing near the launch site in order to be re-flown within hours of the return.
On a side-note at IAC 2016, Elon Musk said the Tanker could function as a Single-Stage To Orbit (SSTO) vehicle, though with negligible payload mass. However, traveling sub-orbital, the prospective vehicle could reach any point on Earth within a 25-minute period with passengers or cargo.