Gaia – Mission Science
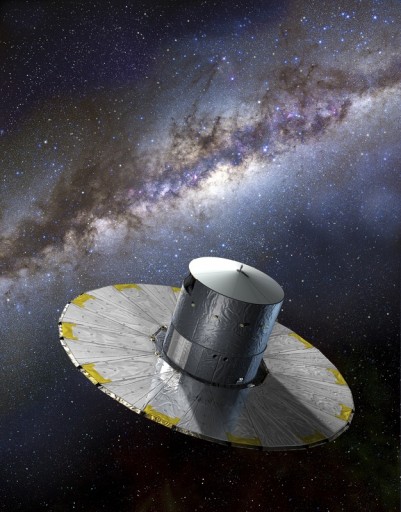
ESA’s Gaia Spacecraft will complete a five-year mission to fulfill the following basic mission objectives:
- determine the astrometric properties (angular position, proper motion, parallax) of one billion stars with an accuracy of 20 microarcseconds at a brightness of 15 mag and 200 microarcseconds at 20 mag
- determine the radial velocity of 150 million stars brighter than 17mag
- detect physical parameters (mass, temperature, chemical composition) of observed stars
- accurate tests of Einstein’s Theory of General Relativity
- detect extra-solar planets, near Earth objects, distant quasars and other objects and determine their positions and motion
Gaia’s core objective is to create the largest, most precise three-dimensional map of the Milky Way Galaxy by surveying more than one billion stars. All objects that are brighter than magnitude 17 will be measured by Gaia’s three instruments: the Astrometric Instrument, Photometric Instrument and Radial Velocity Sensor.
Each object will be studied 70 times over the course of the primary mission – logging their positions, distances, movements and changes in brightness as well as properties such as mass, temperature and chemical composition.
Because of Gaia’s unprecedented sensitivity and accuracy, the mission is expected to discover hundreds of thousands of new celestial objects including extra-solar planets. Gaia also studies asteroids within the solar system and more distant quasars.
Data provided by Gaia will shed light on long-standing questions related to galactic structure and evolution, star dynamics, exoplanets and solar systems. Gaia provides data to identify which stars originally belonged to smaller galaxies that were swallowed by the Milky Way. Watching the large scale motion of a large number of stars can point to the distribution of dark matter throughout the galaxy.
Gaia Milky Way Coverage
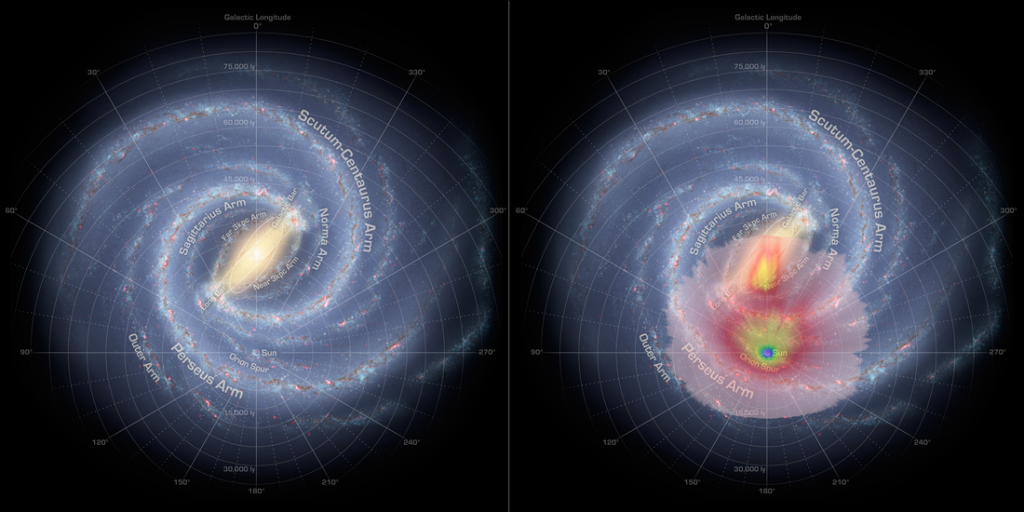
Galactic Structure
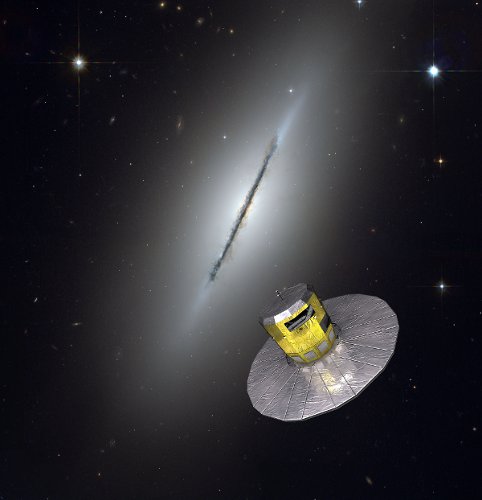
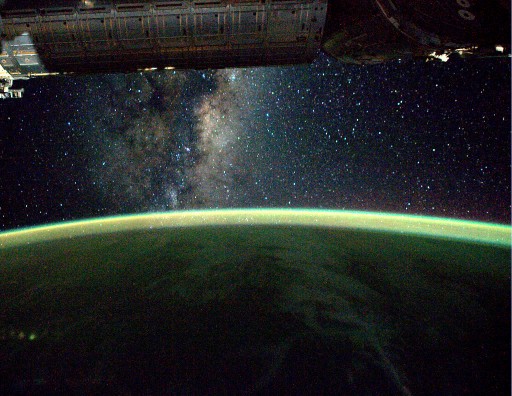
The Milky Way consists of a flat disk that contains ~100 Billion stars of all spectral types and ages that are orbiting around the Galactic center. This disk shows spiral structure and contains interstellar material, mostly atomic and molecular hydrogen as well as dust. Within the disk are open star clusters and super clusters/moving groups of stars. The inner kilo-parsec of the galaxy forms a bulge that is less flattened and is comprised of moderately-aged stars. At the center of the Milky Way is a supermassive black hole called Sagittarius A*. The bulge and central disk are surrounded by a halo of about a Billion old, metal-poor stars along with about 160 globular star clusters. The entire galaxy system is embedded in a matrix of dark material of unknown properties and composition.
The distribution of stars within the Milky Way is linked through gravitational forces as well as zones of star formation. The initial distributions are modified by small and large scale dynamic processes that include instabilities and mergers with other galaxies.
Understanding the Milky Way Galaxy requires measurement of distances and space motions for a large number of stars of different mass, age and metal content. That is exactly what Gaia will accomplish.
The all-sky astrometric survey performed by Gaia will provide data that allows to study the composition, formation and evolution of our Galaxy. Studying a total of one billion stars covers a large portion of the Galaxy’s volume and determining the three-dimensional and radial velocity of all stars allows scientists to determine the origin of the stars – whether they originated within the Milky Way or if they are originally from a separate galaxy that was swallowed by our galaxy.
Determining the color and luminosity of stars points to their age and stellar type. This allows Gaia to create an accurate history of star formation in the Milky Way. The Galaxy’s star formation history will be derived from the observed current distribution of stars over stellar type.
The huge number of stars to be studied allows Gaia to quantify the understanding of the structure and motions within the bulge, the disk, the spiral arms and the outer halo to substantially improve dynamical studies of the Milky Way.
Data obtained from the Milky Way also applies to other galaxies that can not be studied directly.
ESA has provided the following questions that will be answered by Gaia:
- Do large galaxies form from accumulation of many smaller systems which have already initiated star formation?
- Does star formation begin in a gravitational potential well in which much of the gas is already accumulated?
- Does the bulge pre-date, post-date, or is it contemporaneous with the halo and inner disk?
- Is the thick disk a mix of the early disk and a later major merger?
- Is there a radial age gradient in the older stars?
- Is the history of star formation relatively smooth or highly episodic?
Stars & Stellar Evolution
The study of stars and stellar structure and its evolution provides fundamental cosmological data on the evolution of galaxies. Although the fundamental processes of stellar evolution are well understood, there are still a number of theories that require further study.
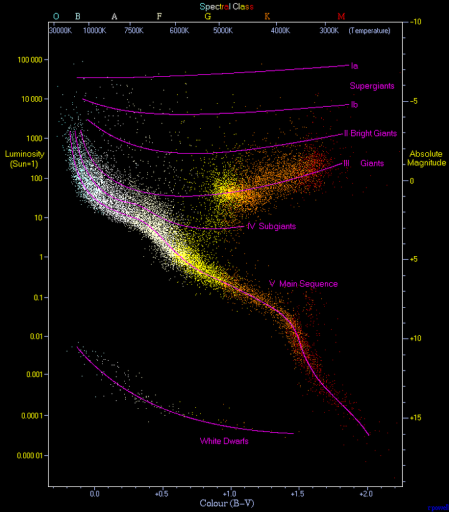
As a star evolves, its internal composition goes through changes due to a number of internal processes leading to changes in properties such as temperature and luminosity. Over the course of stellar evolution, the star’s energy source (the fusion of light elements in its core and shells) progresses through a series of different elements.
Over the course of its evolution, a star follows a track on the Hertzsprung-Russel diagram which plots luminosity against star color. Stellar models that are widely agreed on predict a certain track as a star moves in the diagram, but previous measurements have shown discrepancies from the models.
Gaia will improve models by providing accurate luminosity values, surface temperatures, chemical abundances, stellar masses and other properties to further probe the existing models or create modified theories.
Further work on stellar modeling is required for atmospheric dynamics, transport processes of matter, angular momentum and magnetic fields.
Data that is highly desired includes studies of rare objects such as distant stars and stars undergoing rapid evolutionary processes as well as high quality data of common objects as part of a census across all stellar populations.
Combined with astroseismic data, the accurate global parameters provided by Gaia can help determine the size of the convective cores in order to assess the amount of nuclear material that is available to sustain the luminosity of a star which plays a major role in the evolution of high-mass stars.
Another aspect that Gaia will study is the internal diffusion of chemical elements. The diffusion of chemical elements in stellar radiative zones has important effects on stellar evolution particularly during evolution phases during which the stellar core is provided with fresh helium. Diffusion may also be the driving force behind changes of the composition of the surface of stars over the course of their life. This may be the reasons for the discrepancies in position in the Hertzsprung-Russel diagram between measured values and predicted properties. These discrepancies will be addressed because Gaia samples a huge amount of stars providing accurate positions in the diagram for all of them over a period of several years.
Gaia data will also improve the capability of dealing with non-local convective models for stellar interiors.
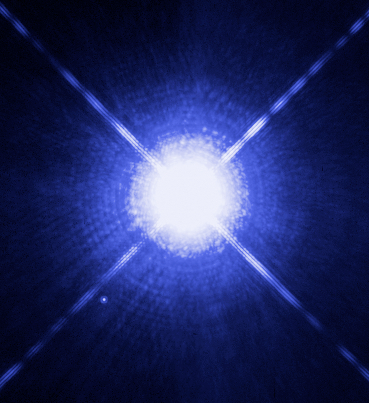
White Dwarfs
Gaia will be looking at White Dwarfs to answer a number of important questions related to stellar and galaxy evolution. White Dwarfs are created when a low-mass star can no longer sustain nuclear fusion in its interior that leads to the collapse of the stellar core resulting in a compact structure.
As White Dwarfs no longer generate radiation, they cool down over a period of several Billion years – progressively decreasing in luminosity. Gaia will look at White Dwarfs in the 10-parsec environment of the solar system to determine the age of the solar neighborhood by studying the cooling process of White Dwarfs. Galactic evolution will be studied by observing different populations of White Dwarfs and determining their age.
Right: Sirius A in the center and Sirius B, a White Dwarf, as a faint dot next to it.
Brown Dwarfs
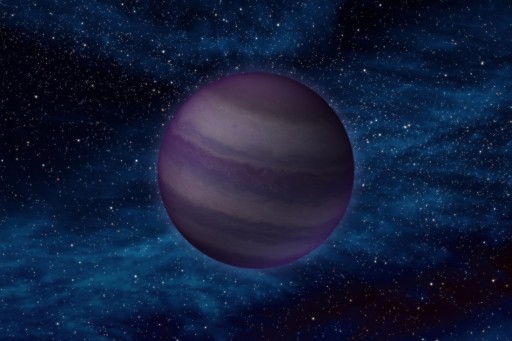
A Brown Dwarf is too low on mass to sustain Hydrogen Fusion reactions in its core. They have masses that range between twice the mass of Jupiter and the lower mass limit for nuclear reactions. Because of the lack of Hydrogen fusion, Brown Dwarfs are dim and cool when being compared to stars. Although Brown Dwarfs do not emit visible radiation, they still radiate heat in the form of infrared and near infrared radiation.
Brown Dwarfs are very abundant , but their formation processes, intrinsic properties and properties of populations of Brown Dwarf are poorly understood. First discovered in 1995, Brown Dwarfs have proven hard to study because of their extremely low luminosity. Brown Dwarfs often accompany main-sequence stars, forming a binary system. Mostly, M-Type stars feature these companions. Gaia will observe about 50 million M-Type stars which will enable the spacecraft to gather data on a large number of binary systems containing a Brown Dwarf.
Gaia aims to detect these systems as separated components and answer questions on the origin of free-floating Brown Dwarfs, the formation process of Brown Dwarfs in binaries and the binary distribution characteristics such as mass ratio and separation between the star and its Brown Dwarf.
Brown Dwarf Binary
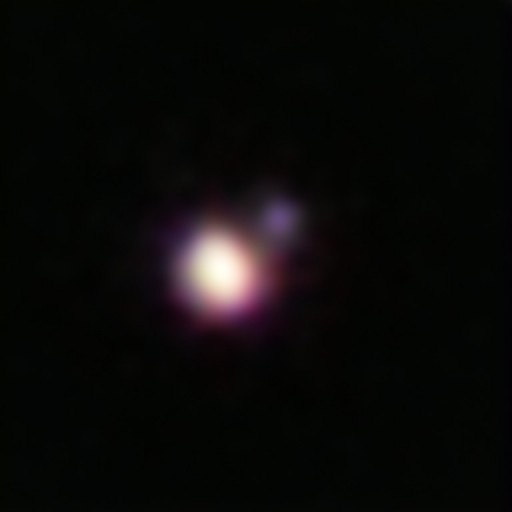
Variable Stars & Binaries
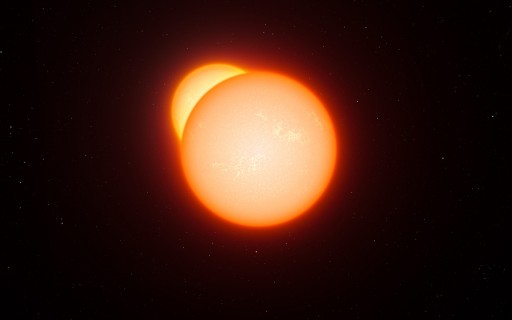
Gaia is capable of tracking changes in brightness over the entire visible spectrum and a portion of the near-infrared range using its photometric instrument. Stars can exhibit changes in brightness on short scales of a few seconds or large scales of years. Gaia can pick up short-term changes and changes on a longer scale of up to five years. These changes allow the observatory to detect classic periodic variables, eclipsing binary systems, Cepheids and other phenomena of stellar evolution.
Gaia will resolve all binaries with distances greater than 20 microarcseconds observing binaries with orbital periods between hours and millions of years. Tens of millions of binaries will be sampled by Gaia, but due to the large distances of most binaries, orbit determination will only be possible in few cases. Semi-major axis and mass ratio will be deduced by separation distribution and magnitude differences.
For binaries with orbits of 0.03 to 30 years, Gaia will be able to recognize the binaries as short period systems.
Periods of 7 to 8 years allow Gaia to determine a photocenter orbit. For brighter systems, radial velocity measurements can be used to define shorter-period binaries.
Supernovae
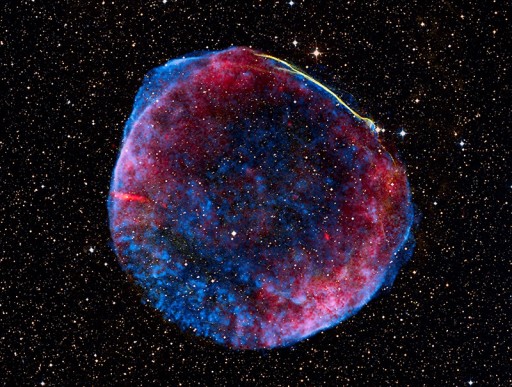
Gaia will study nearby supernovae to generate a high-quality data set of Type-Ia supernovae. Because of the size of the data set, Gaia is expected to provide information on rare phenomena such as Type-Ib and Ic supernovae.
Type I supernovae are subdivided in three groups based on their spectral signature. Type Ia supernovae show a strong ionized silicon absorption line while Type Ib shows strong neutral helium lines. Type Ic lacks the silicon and helium lines.
Gaia will also study Type II supernovae that show broad emission lines.
In total, Gaia will observe more than 21,000 supernovae. Astrometric measurements will provide the precise position of the supernovae providing scientists with the opportunity to identify progenitors in nearby galaxies and for studying the spatial distribution of supernovae in different types of galaxies.
Distance & Age Scales
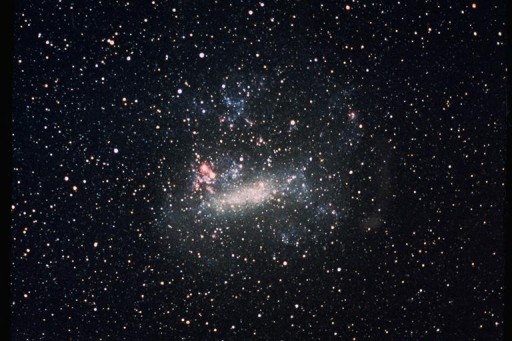
Gaia will provide distances and physical parameters for all types of observable objects in the Milky Way and neighboring galaxies to provide the opportunity to assess distance scales in the Universe. The Hubble Constant is a major cosmological parameter that describes the rate of expansion of the universe. It was determined by tracking distance indicators of a number of galaxies and stars including the Large Magellanic Cloud LMC and the Cepheids. As these distance measurements come with a large uncertainty, there is some doubt to the accuracy of the Hubble Constant which currently stands at 74.3km/s*Mpc with an error margin of 2.1.
Gaia will use its measurement techniques to track the distances to the Cepheids and bright stars within the LMC to provide accurate measurements that could improve the accuracy of the Hubble Constant. The second fundamental parameter that Gaia will try to quantify is the age of the Universe. This will be accomplished by determining the distances of sub-dwarf stars and globular clusters that are the oldest objects in the galaxy. Using theoretical models, the measured distance to these objects will lead to a good estimate of their age and with that the age of the Universe.
Solar System
In addition to distant stars in the Milky Way, Gaia will track and study all solar system objects brighter than 20mag. These objects include asteroids and comets in orbit around the sun.
Gaia will track the positions and velocities of asteroids over the course of its five year mission allowing scientists to determine their orbit. Also, Gaia is capable of determining the mass of objects by making astrometric measurements. As two small objects make close encounters, the gravitational pull they have on each other slightly alters their trajectory which will be recorded by Gaia. The mass of the objects can be derived from this data.
Photometric observations of small solar system objects provides data on their surface properties and composition.
Gaia will observe the main belt asteroids located between Mars and Jupiter. Also, several thousand Near-Earth Objects that cross the orbit of Earth will be discovered and characterized by Gaia. The observatory can observe to an angular distance of 45° from the Sun allowing it to look into a blind spot that is unobservable from the Earth. Gaia is expected to detect a wealth of objects that orbit between the sun and Earth. Objects in exotic orbits far from the ecliptic are also expected to be found.
Gaia can see what Ground Based Observatories can’t
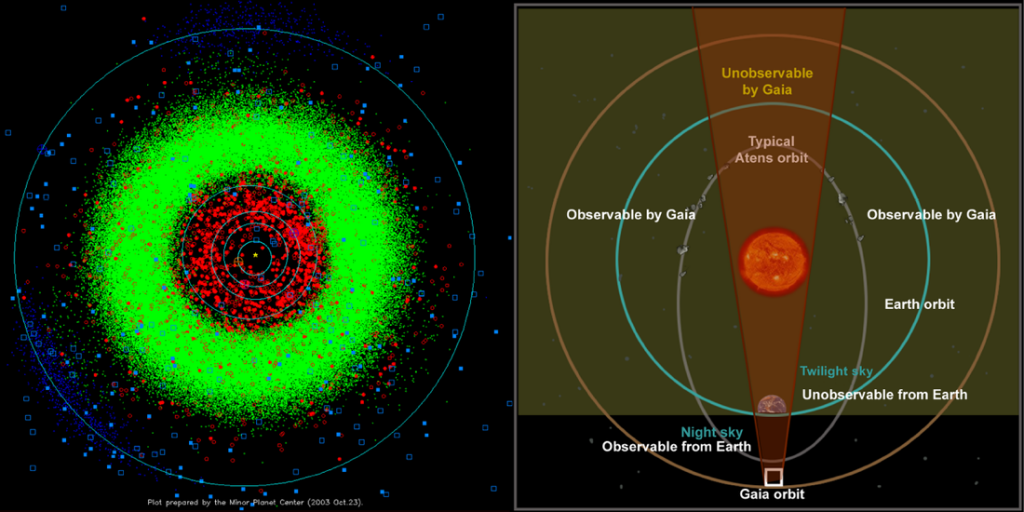
Trojans
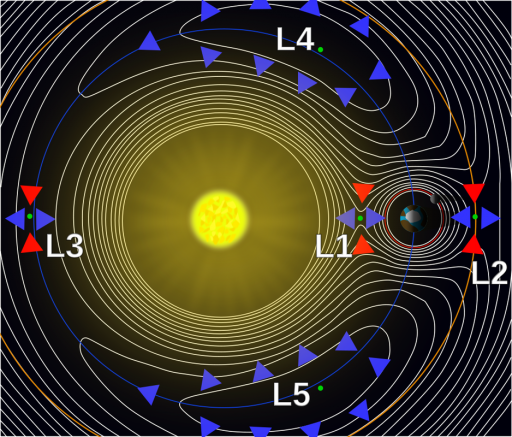
In each planet-sun system, there are five Lagrangian Points which are locations where the combined gravitational field of the two bodies provides precisely the force for a small object to orbit with the system. Objects in these points maintain their position relative to the other two objects indefinitely unless being disturbed by another force. Gaia itself is making use of the L2 point in the Earth-Sun system, but the observatory will detect objects in other Lagrangian Points of other planet-sun systems.
Objects that are located in Lagrangian points 4 & 5 and orbit the sun together with a planet are known as Trojans. These types of objects have been discovered in the Neptune-Sun system and Jupiter-Sun system.
Gaia will precisely determine the orbits of Trojans and allow scientists to deduce whether the objects were formed where they are found today of if they were trapped at some point. Photometric measurements of large Trojans will reveal their rotation periods, spin axis orientation and shapes & sizes. Additionally, spectroscopy will be used to determine the chemical composition of large Trojans.
Gaia will also look at the distribution of Trojans and study if there are any differences between the L4 and L5 points with respect to the amount of Trojans and their orbits around those points.
Jupiter Trojans
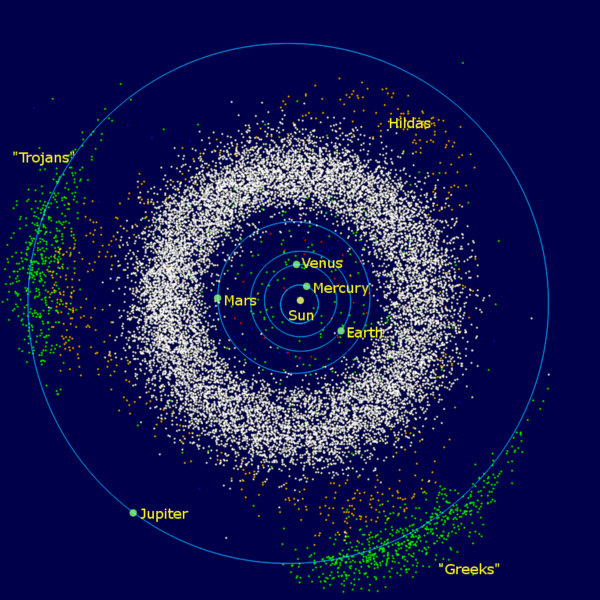
Trans-Neptunian Objects & Centaurs
As part of its observations, Gaia will track small solar system objects that come no closer to the sun than Neptune, having their perihelion between 5.2 and 30 astronomical units. These objects are called Centaurs. Further away from the sun is the Kuiper Belt of icy objects that will also be monitored by Gaia.
Because these objects are very small and far away, they appear very faint and most will not be picked up by Gaia because of the 20mag detection limit. Nevertheless, Gaia will be able to track large Trans-Neptunian Objects to determine their orbits and sizes as well as binarity as most objects in this region are of binary nature. Of special interest are high-inclination objects that can not be observed from Earth.
Kuiper Belt Objects
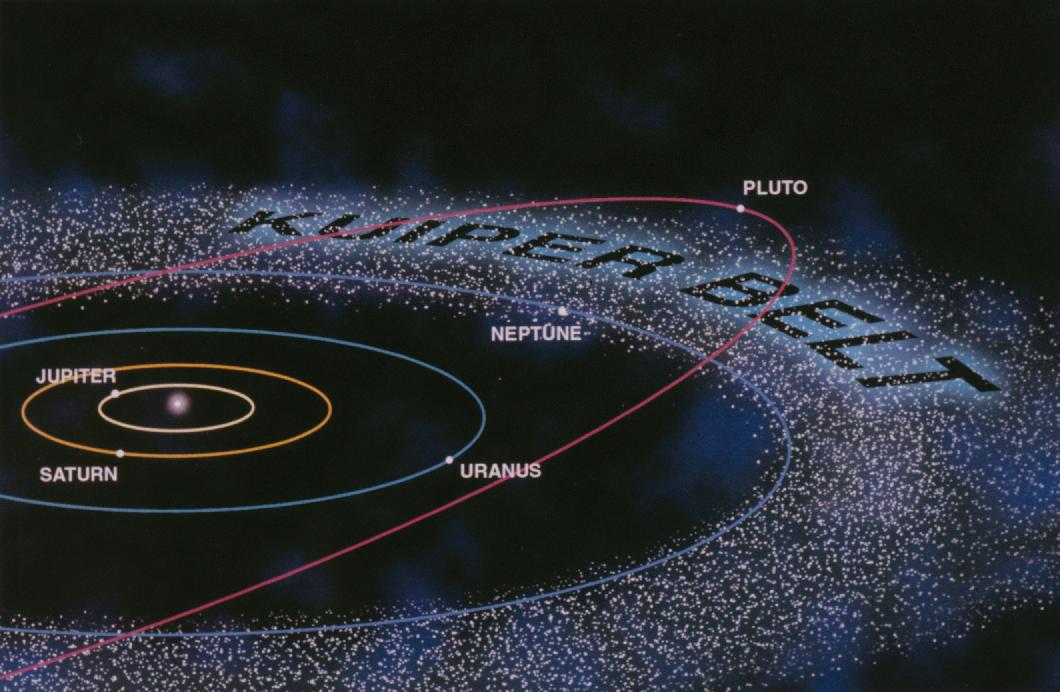
Oort Cloud
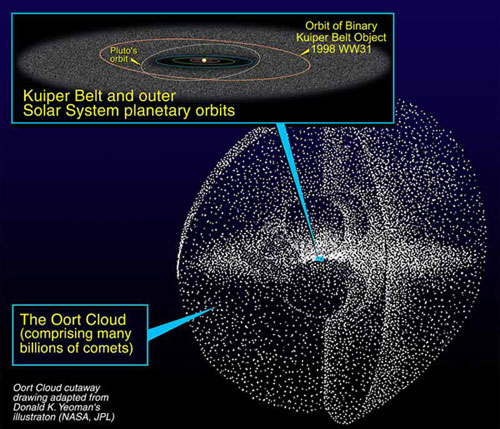
Far beyond the orbits of the outer planets, between 1,000 and 150,000 astronomical units, lies a hypothesized spherical cloud of icy bodies called the Oort Cloud. No direct observations of the Oort Cloud have been made to date, but the general believe is that long-period comets such as the famous Comet Halley have originated from the Oort Cloud. Halley-type comets have to be replenished constantly as their lifetime is much shorter than the existence of the solar system.
Observations of Halley-type comets have revealed that they have similar aphelion distances, an isotropic distribution of orbital inclinations around the sun and an isotropic distribution of the direction of the perihelion points. These observations have led to the theory of the spherical Oort Cloud as there is no preferred direction from which these comets enter the inner solar system.
Because the Oort Cloud (if present) has to be of considerable size it is susceptible to close passages of stars that deflect comets into the solar system. These comets are the cause of Earth-crossing comet showers and possible impacts.
Gaia will complete a detailed survey of the stars in the neighborhood of the Oort Cloud in order to determine the frequency of such close encounters. The observatory will study all star systems within 50 parsec from the Sun and precisely calculate star positions and motion that can be used to calculate any past and future encounters. This data will show whether the currently observed number of comets is and average or if we are currently seeing a period of enhancement which will allow scientists to assess the population of the Oort Cloud.
Exoplanets
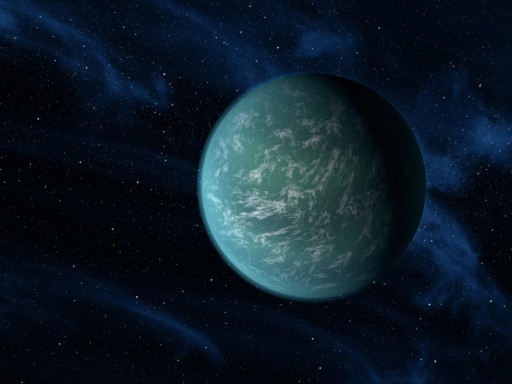
The search for Exoplanets has been one of the most important fields of activity over the past several years. Gaia will be studying a huge number of stars over the course of its mission and is expected to detect thousands of extra-solar planets using an astrometric and photometric approach.
As a planet orbits its star, it induces a wobble motion as the star also orbits around the center of gravity of the combined system. Gaia’s precise astrometric measurements will be sufficient to detect long-period planets over a number of measurements over the primary mission.
Exoplanet systems with the proper orbital alignment relative to the observer can be detected by looking for transits of an exoplanet. As the planet traverses across the disk of its parent star, the overall brightness of the star is reduced – depending on the size and orbit of the planet. Gaia will see many transit events. Detailed observations will be possible for stars of 16 mag or brighter. Gaia operates at a millimagnitude accuracy allowing it to detect Jupiter-sized planets around stars down to 14 mag.
Measurements will provide estimates of planet masses which allows scientists to assess whether dynamical interactions in any given extra-solar planet system would permit an Earth-like planet in the habitable zone.
Gaia Accuracy
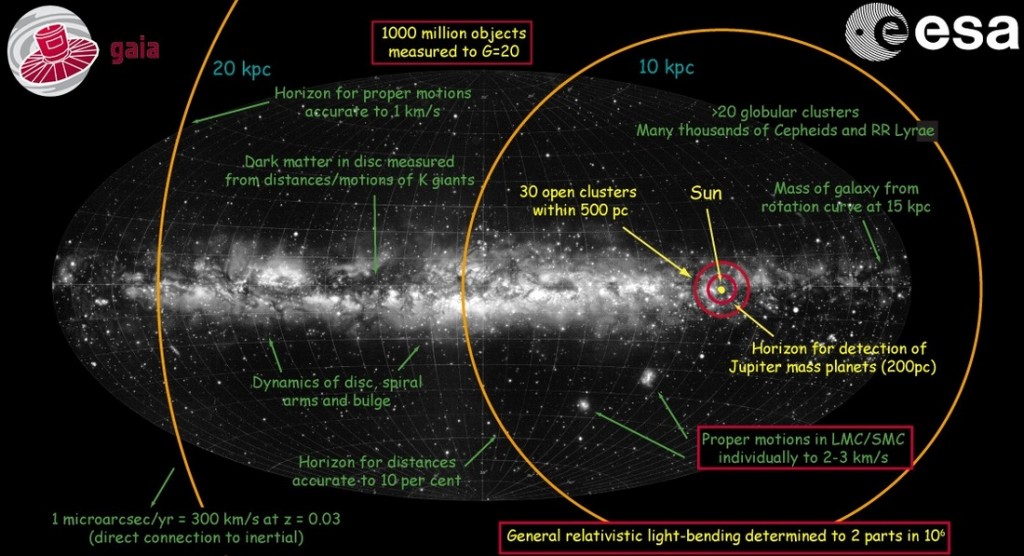