Gaia Mission & Orbit Design
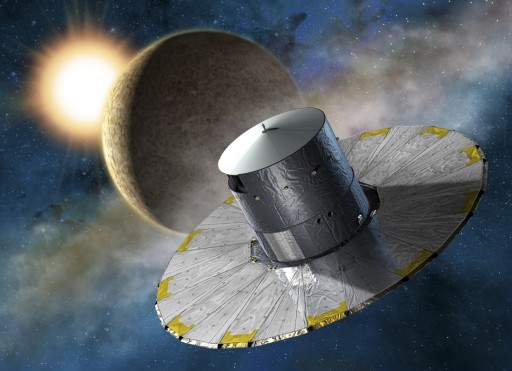
The Gaia mission sets out to perform a five-year primary mission to generate a three-dimensional map of the sky, tracking more than one billion stars to determine their position, angular and radial velocity, mass, temperature, chemical composition and other properties. Gaia operates from an orbit around the Sun-Earth Lagrange Point 2 after being sent there by a Soyuz/Fregat launch vehicle.
Pre-Launch
The Pre-Launch Mission Phase begins with the installation of the Gaia spacecraft on the Soyuz Launch Vehicle. Prior to that, Gaia completes final preparations at the launch site, including testing, propellant loading and final reconfigurations to put the observatory in its launch mode.
Once atop the Soyuz Launch Vehicle, the integrated stack completes a number of countdown and flight rehearsals to verify the flight software and allow launch team members to exercise procedures. Additionally, Gaia is going through final pre-launch reconfigurations such as battery charging.
Launch, Ascent & Insertion
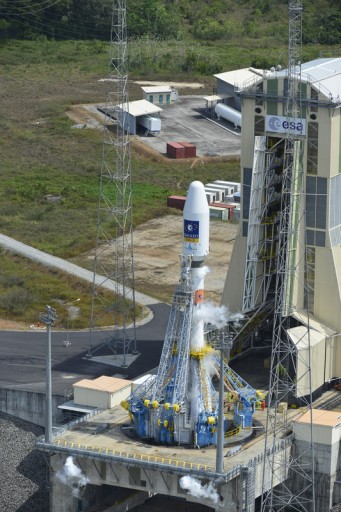
ESA’s Gaia Spacecraft is launched on a Soyuz 2-1B Rocket with a Fregat Upper Stage blasting off from the ELS Launch Site at the Guiana Space Center, French Guiana.
Soyuz 2-1B is one of two modernized versions of the workhorse of the Russian & Soviet Space Programs. It is nearly identical to previously flown Soyuz launchers, but features an upgraded Control System switching from analog to digital control systems to make the Soyuz Launcher more flexible, and an upgraded second stage featuring the RD-0124 Engine.
Soyuz 2-1B features a large Core Stage with a single four-chamber RD-118 engine, four strap-on Boosters each with its own RD-117 engine and a third stage featuring an RD-0124 staged combustion engine. All stages of the Soyuz use Liquid Oxygen as oxidizer and rocket-grade Kerosene as fuel. For the Gaia launch, Soyuz uses a Fregat upper stage to perform multiple burns to inject the payload to its desired trajectory. The stack is topped by a 4.1-meter payload fairing.
Overall, Soyuz stands 46.1 meters tall with a core diameter of 2.95 meters, a span of 10.3 meters and a liftoff mass of 308,000 Kilograms. See our detailed launch vehicle overview for all details on the Soyuz 2-1B rocket and Fregat Upper Stage:
The Launch Phase starts with the initiation of the Soyuz Countdown eight hours before liftoff. Early Countdown Operations include the installation of batteries in the boosters and preparations for propellant loading that starts four hours before launch.
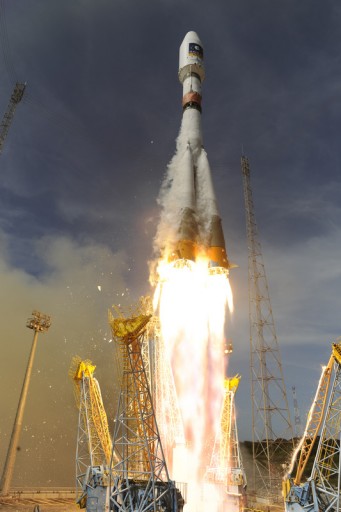
The four boosters, core stage and second stage are loaded with a total of 198,200 Kilograms of LOX and 82,300kg of Kerosene over a period of 2 hours and 15 minutes. Fregat is loaded with hypergolic propellants before integration with the payload. After fueling and final launch system tests, the rocket is exposed by retracting the Mobile Service Structure at L-60 minutes. Six minutes before liftoff, the Soyuz Automated Countdown Sequence starts during which propellant tank pressurization and transfer to internal power as well as final Guidance System Configuration are performed. Engine ignition comes 20 seconds before liftoff to allow all engines to build-up thrust before vehicle release and liftoff at T-0.
Blasting off with a total liftoff thrust of 425,000 Kilograms firing the four RD-117 and single RD-118 engine, Soyuz begins its ascent with a short vertical climb before pitching and rolling onto its pre-planned flight trajectory. Each of the four boosters of the Soyuz is 19.6 meters long, 2.68m in diameter holding 39,200kg of propellants that are expended during a 1-minute 58-second burn. Upon burnout of the Boosters, they are separated, headed for a crash landing 350 Kilometers downrange in the Atlantic Ocean.
After Booster separation, the Core Stage continues to fire its RD-118 engine providing 102,000 Kilograms of vacuum thrust. The Core is 2.95 meters in diameter and 27.8 meters long with a propellant load of 98,500 Kilograms used up over the course of a 4-minute 45-second engine burn. Soyuz uses a hot-staging technique: Two seconds after Core Stage Cutoff, the third stage ignites its RD-0124 engine and the separation system is initiated at the same time allowing the exhaust of the third stage to push the spent core stage away.
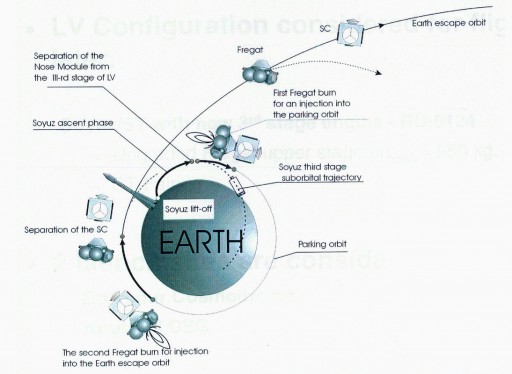
The 3rd stage of the Soyuz is 2.66 meters in diameter and 6.74 meters long holding 25,400 Kilograms of propellants that are consumed by the RD-0124 engine to provide 30,000 Kilograms of vacuum thrust. RD-0124 completes a 4-minute 20-second burn to deliver the stack to a sub-orbital trajectory. Third stage cutoff occurs at T+9:15 and the Fregat Upper Stage along with the Gaia Payload separate at T+9:23 to continue the flight.
After a very short coast period of just one minute, the Fregat Upper Stage ignites its S5.92 engine to boost the stack into a Parking Orbit. Fregat is 3.35 meters in diameter and 1.5 meters long capable of holding 5,350 Kilograms of Unsymmetrical Dímethylhydrazine fuel and Nitrogen Tetroxide oxidizer. Fregat is an autonomous Upper Stage that is equipped with its own power, propulsion and control system to perform flights of up to 48 hours. S5.92 is operated in two thrust modes – 2,025 Kilograms and 1,430 Kilograms.
The first Fregat Upper Stage Burn boosts the stack into a Low Earth Parking Orbit of 175 Kilometers and an inclination of 14.98 degrees. After nearly one revolution of Earth, Fregat re-ignites and boost the stack into the hyperbolic L2 Transfer trajectory. Following the completion of the second Fregat burn, Gaia is separated and enters its transfer mode. Fregat makes another maneuver to enter a graveyard orbit to avoid re-contact with Gaia.
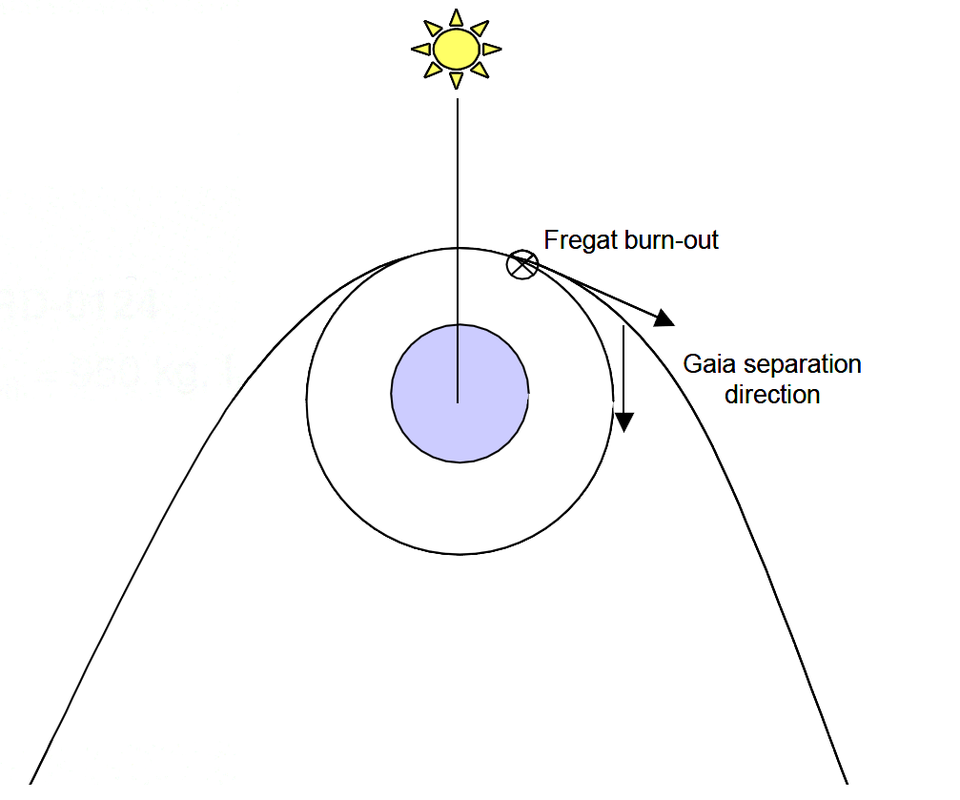
L2 Transfer & Orbital Insertion
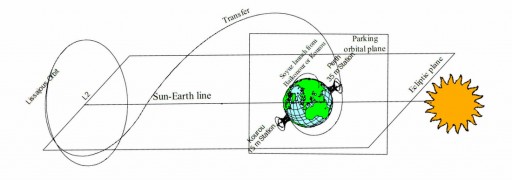
Following its launch atop the Soyuz/Fregat vehicle, Gaia enters a hyperbolic L2 Transfer Trajectory that allows the vehicle to reach the Sun-Earth Lagrange Point 2 in 3 weeks. As part of initial operations in space, Gaia starts establishing communications with ESA ground stations and begins a series of steps to acquire three-axis control. While the sun shield is still in its stowed position, Gaia can only use its gyros and –X facing thrusters to enter a stable attitude. Early in the mission, the Deployable Sun Shield is deployed.
The DSA is a umbrella-type structure that consists of Multilayer Insulation as the primary shield material and six rigid deployment booms as well as six secondary stiffeners. These booms have a single articulation on the base of the Service Module for easy deployment in the radial direction by a spring system. Spacing cables link the booms to the others to ensure a synchronized deployment sequence. The DSA is 10 meters in diameter.
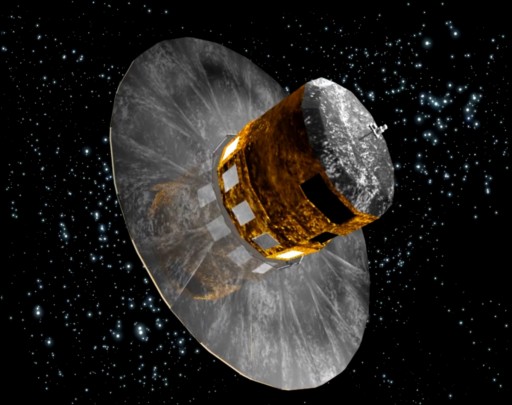
When the DSA is deployed, Gaia starts receiving power from its six solar panels that are installed on the Deployable Sunshield Assembly. Verifying that Gaia is power-positive is an important step in the mission. Gaia’s Launch and Early Orbit Operations Phase is expected to last about two days featuring basic vehicle health and status checks.
Using ranging techniques, the precise trajectory of the Gaia spacecraft is determined over the first two days of the flight to assess the accuracy of the insertion performed by the launch vehicle. If necessary, Gaia performs an initial Trajectory Correction Maneuver as early as L+2 Days followed by another maneuver to correct leftover bias by Flight Day 12.
Over the course of the L2 Transfer, Gaia begins spacecraft commissioning operations.
These procedures include extensive electrical testing of all spacecraft systems and activations of the instruments to put them through a complete checkout.
Throughout the transfer, Gaia is spin-stabilized. After covering the 1.5 Million Kilometers to the L2 point, Gaia uses its Chemical Propulsion System to perform its Orbit Insertion Maneuver – a 180-meter per second burn that places the vehicle in a Lissajous Orbit around the L2 Libration Point. Two Days after insertion and orbit determination, an orbit correction maneuver is performed to place the vehicle in its desired orbit.
L2 Location
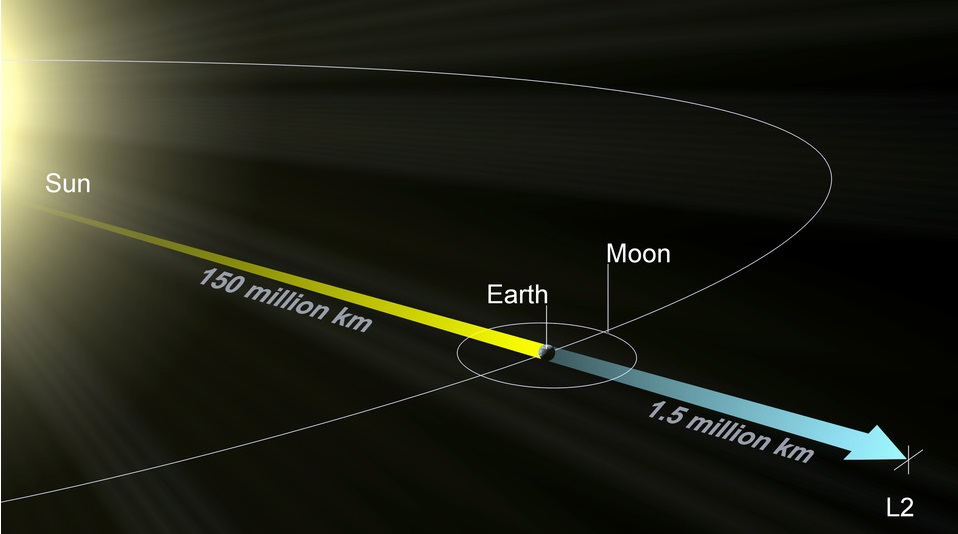
Orbit Design & Scanning Law
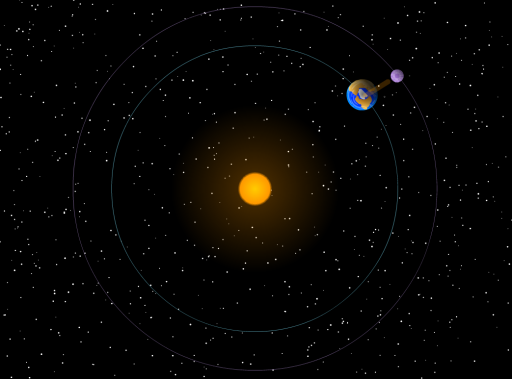
The L2 Lagrange Point offers a semi-stable gravitation environment that allows spacecraft to reside in orbits around the libration point over a period of years at the expense of few propellants for orbit maintenance. Since the Sun, Earth and Moon are behind the field of view, the L2 Orbit offers a high observation efficiency as well as a stable thermal environment and a low radiation dose.
A Lissajous Orbit describes a Lissajous Curve around the Libration Point with components in the plane of the two primary bodies of the Lagrange System and a component perpendicular to it. The orbit period is about 180 days and the size of the orbit is 263,000 x 707,000 x 370,000 km.
In theory, Lissajous orbits are highly stable, but in practice, an orbit around a Libration Point is dynamically unstable – requiring some effort to model as small departures from equilibrium grow exponentially as time progresses. These departures require regular orbit maintenance maneuvers to preserve a stable orbit within mission parameters. The progression of a Lissajous Orbit is largely dependent on its initial parameters – namely phase angle and distance to the L2 point.
Gaia Orbital Plots
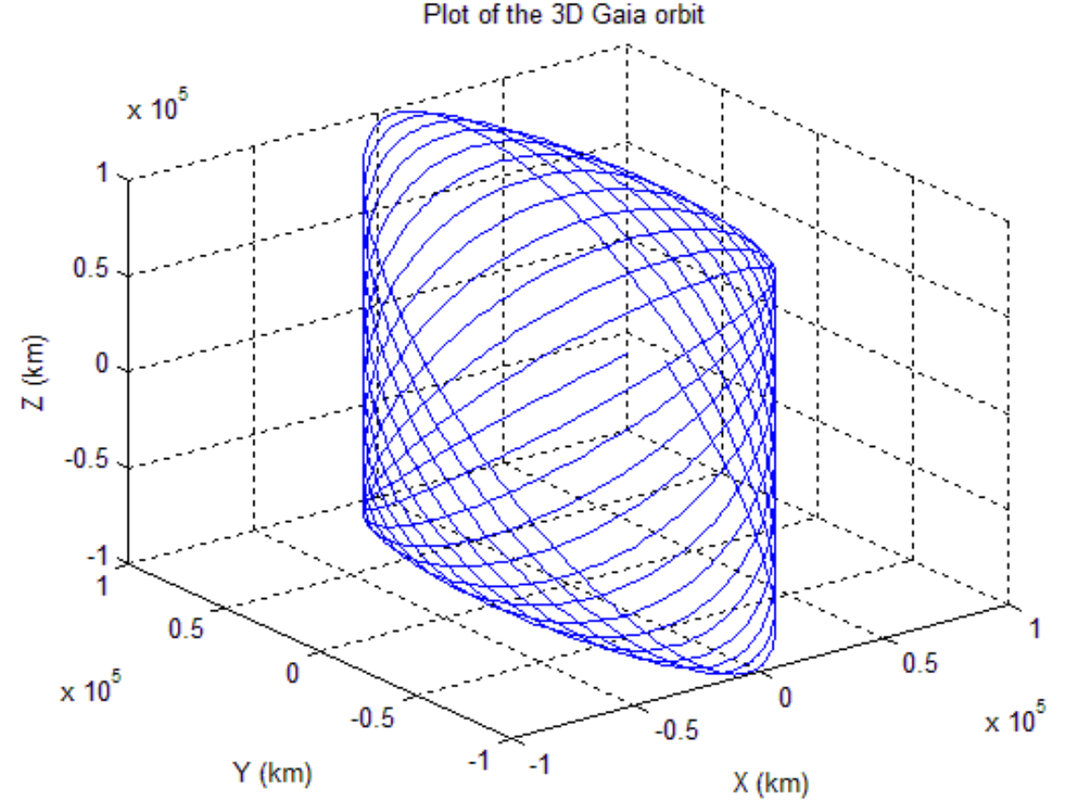
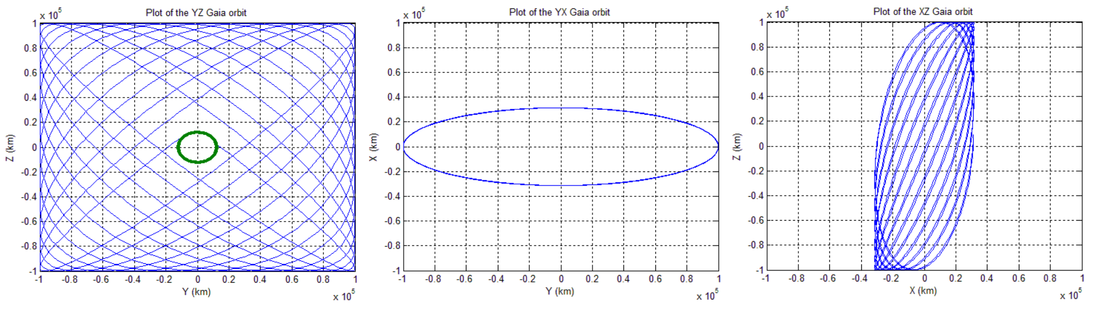
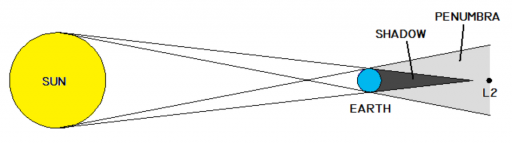
These parameters have to be set and controlled very carefully in order to meet the mission requirements for the Gaia mission. The exact orbital parameters around L2 are not relevant to the Gaia mission as long as proper tracking and position information are provided for data analysis. The biggest constraint for the Gaia mission, and the most important aspect of orbit design, is solar illumination. As the L2 point is located “behind” Earth and rotates along with Earth, the planet constantly casts a shadow at a spherical zone of 13,000 Kilometers.
Gaia can not afford to enter eclipse during its mission because it would cause a significant thermal shock to the system that would render the observatory useless. That is why Gaia has to avoid the Penumbra Zone for the duration of the science mission and extended mission. The initial orbital parameters of Gaia have been chosen so that the Lissajous Orbit evolves in a way that avoids a solar eclipse for 6.3 years which is long enough for the primary and extended Gaia mission. Placing the Gaia spacecraft close to the eclipse zone during insertion prevents the occurrence of an eclipse during the mission, the father away the initial position is from the Penumbra Zone, the quicker an eclipse occurs in the mission.
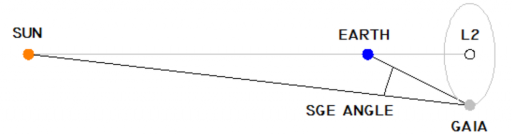
Another aspect is the Sun-Gaia-Earth Angle that can not exceed 15 degrees for observation purposes. This requires a precise selection of the amplitudes of the Lissajous Orbit. After the initial parameters of the orbit are achieved, the angle progresses in an oscillatory convergent motion up until a point halfway through the science mission after which it evolves in an oscillatory divergent motion until the end of the mission.
Modeling has shown that the angle will be within 0.4 to 5.3 degrees for the target orbit which is well within requirements.
Simulating the Lissajous Orbit and its progression is already a complex task, but for modeling of the scanning law other motions have to be taken into account such as the orbit of the L2 point around the sun, the rotation of the Gaia spacecraft itself and the precession of its spin axis following a circle around the Gaia-Sun line.
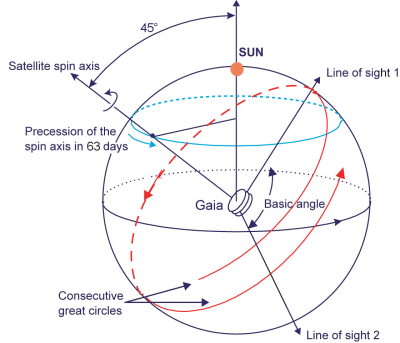
Gaia follows a revolving scanning law. The observatory spins along its axis at a speed of 60 arcseconds per second which corresponds to one revolution every six hours. The spin axis is perpendicular to the field of view of the two telescope apertures which allows them to scan across the sky – completing one “great circle” over the period of six hours. The exact spin rate is measured by onboard sensors and is important for the image read-out times on the focal plane because integration times have to be adjusted to microsecond accuracy based on actual spin rates.
In order to cover the complete sky and not the same great circle over and over again, the spacecraft spin axis has to be in motion as well. The spin axis is in a slow precession motion that is optimized to achieve a maximum angle between spin axis and the sun and to achieve a uniform sky coverage over the science mission. The higher the angle between the spin axis and the sun is, the more accurate the astrometric measurements made during the mission will get. (Higher angles lead to larger measurable parallaxes because the parallactic displacement of transiting stars is proportional to sin ξ.)
This angle ξ is limited by thermal and power constraints. Gaia’s spin axis slowly precesses around the solar direction along a 45-degree angle completing one circle every 63 days – which allows each object in the sky to be observed about 70 times over the course of the primary mission.
The slow precession rate allows Gaia to map subsequent great circles with sufficient overlap to ensure an all-sky coverage is achieved.
Science Mission and Gaia Mission Operations
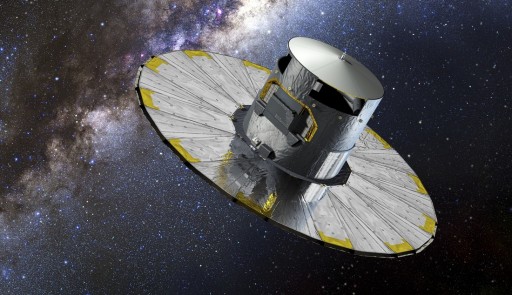
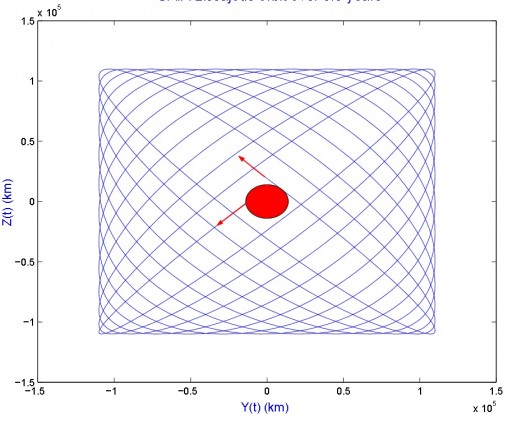
Once in its Lissajous Orbit around L2, Gaia completes three months of commissioning activities that include test observations and calibration activities as well as testing of ground infrastructure with respect to data downlink, processing and archiving.
After these three months, Gaia starts its five-year primary science phase with operations and science data acquisition around the clock for the entire science mission. Spacecraft outages are budgeted for less than 2% of the science mission.
Routine mission operations will consist of a daily science data dump of eight hours as Gaia uses its X-Band Communications System to send stored science data to ESA ground stations in Cebreros, Spain which features a 35-meter antenna, and ESA’s New Norcia station in Australia that also has a 35-meter dish. These passes also feature command uplink if required. The exact scanning law plan is expected to be uplinked once per week to account for densely populated sky regions.
Once per month, Gaia has to perform orbit maintenance procedures which will be small engine maneuvers to make sure orbital parameters around L2 stay within predicted models. These maneuvers are performed without changing the spacecraft attitude so that the scanning law is not interrupted and science operations can continue.
A one time event in the mission is an eclipse avoidance maneuver that could be performed early after orbit insertion depending on insertion parameters or at the half-way mark in the science mission.
The Gaia science mission has a duration of five years with a possible extension of one year and is limited a) by the occurrence of an eclipse, and b) by the onboard supply of bipropellant and Nitrogen MPS propellant.