Space Debris Sensor
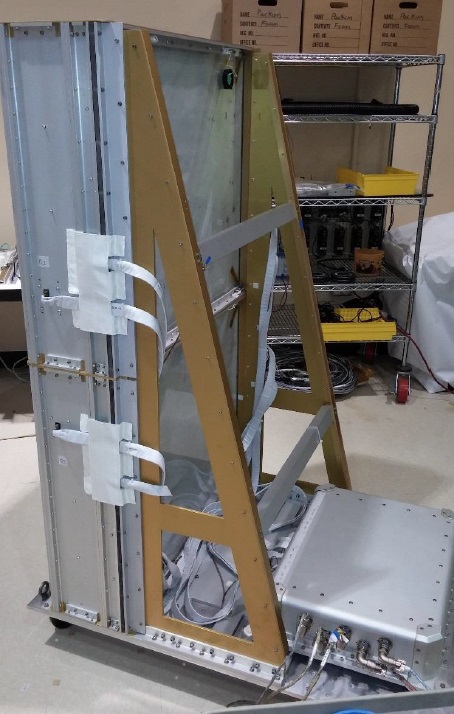
The Space Debris Sensor SDS is a flight demonstration of a novel impact sensor designed to detect and characterize impacts of small debris objects on the International Space Station. It is the first flight demonstration of the Debris Resistive/Acoustic Grid Orbital NASA-Navy Sensor (DRAGONS) developed by the NASA Orbital Debris Program Office.
The core goal of the five-year mission is evaluating two methods for the detection of micro-debris and further characterization with respect to size, speed, direction and density of the incoming objects.
Characterizing the micro-debris environment in different orbital regimes has become an important objective to inform mission designers of the future as sub-millimeter debris are impossible to track from the ground but still can pose risks to satellites.
The existing data on sub-millimeter debris comes from surface analysis on returned spacecraft such as returning Space Shuttles after mission durations of around two weeks, exposure experiments run at the International Space Station and NASA’s Long Duration Exposure Facility that spent nearly six years in orbit between 1984 and 1990. However, this data has been of limited use since the Space Shuttles only flew in a narrow orbital regime and samples returned from ISS show the total accumulated micro-debris strikes over missions of months to years which reduces the accuracy of time and location of debris populations.
SDS can provide data on micro-debris at high temporal resolution, measuring impacts as they happen and providing valuable auxiliary data like debris size and flight direction. A continuous sampling capability is considered critical when attempting to understand the dynamic evolution of the LEO debris environment and SDS, through at least two years of continuous operation, is expected to shed significant light on the population of debris 50 to 500 micrometers in size.
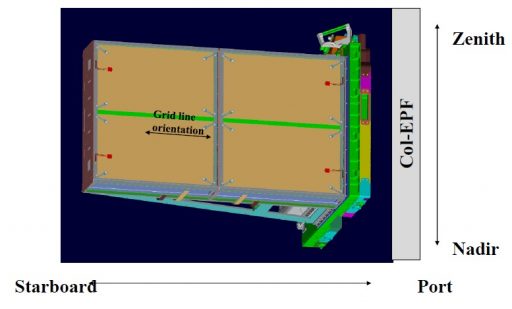
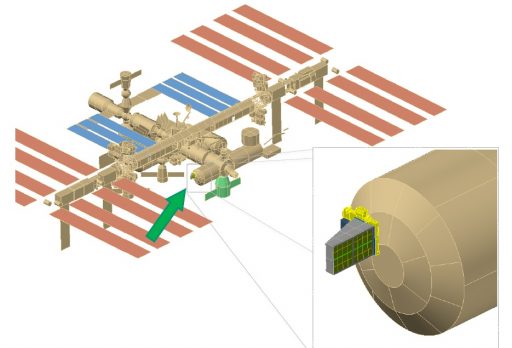
Estimates for the micro-debris population predict approximately 150 million debris pieces larger than one millimeter in orbits between 400 and 1,000 Kilometers, capable of striking orbiting satellites with relative velocities up to 14 Kilometers per second, depending on the collision geometry. At these speeds, even a millimeter-sized particle can pack a fatal punch.
SDS has a total active sensor area of around one square meter and will reside on the forward-facing side of the Space Station, outside the Columbus Module’s External Payload Facility (Starboard Overhead X) so that the detector area can face the ram direction where the probability of debris strikes is highest. The detection principle involves three stages – the first is a resistive grid where the debris size can be determined through the number of conductive wires severed by a strike; the second is a time of flight section to determine the speed of debris; the third is a backstop where the particle is brought to rest to determine its energy. Multiple acoustic impact sensors are attached to each layer to record impact events for time of flight and energy measurement.
The power of SDS resides in the combination of a number of measurement techniques. A well established method used in various smaller debris sensors is the use of a line array of conductive strips of a specific size and specific spacing which, when struck by micro-debris, will be severed, causing a loss of conductivity of one or more wires depending on the size of the debris particle. This will provide the time of the debris event as well as the size of the debris particle by looking at the number of severed strips, either through individual measurement of conductivity through each strip or the overall resistance of the grid panel (SDS uses the latter).
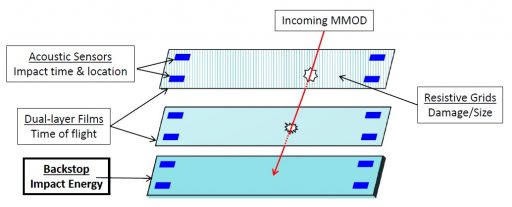
After penetrating the first film, a debris particle will travel 15 centimeters before striking the second layer. Acoustic sensors on both thin films provide the start and stop signals for the time of flight measurement to allow the debris velocity to be calculated. Simple triangulation using the signals from multiple acoustic sensors on each film will yield the impact position on the two layers from which the trajectory of the particle can be reconstructed. The acoustic sensors residing on the backstop deliver the impact kinetic energy.
With the energy and velocity known, the mass of the impact particle can be calculated through E = ½ mv2. The density of the object is estimated by assuming the particle to be spherical with the diameter determined from the hole size on the first layer.
The resistive lines on the first film are 75 µm wide and a 1 Hz data stream logs grid resistance and temperature, allowing events to be pin-pointed to the second they occur through the grid resistance alone. The acoustic sensors log data at 500 kHz when an impact occurs. SDS employs a 5-centimeter Lexan plate as backstop.
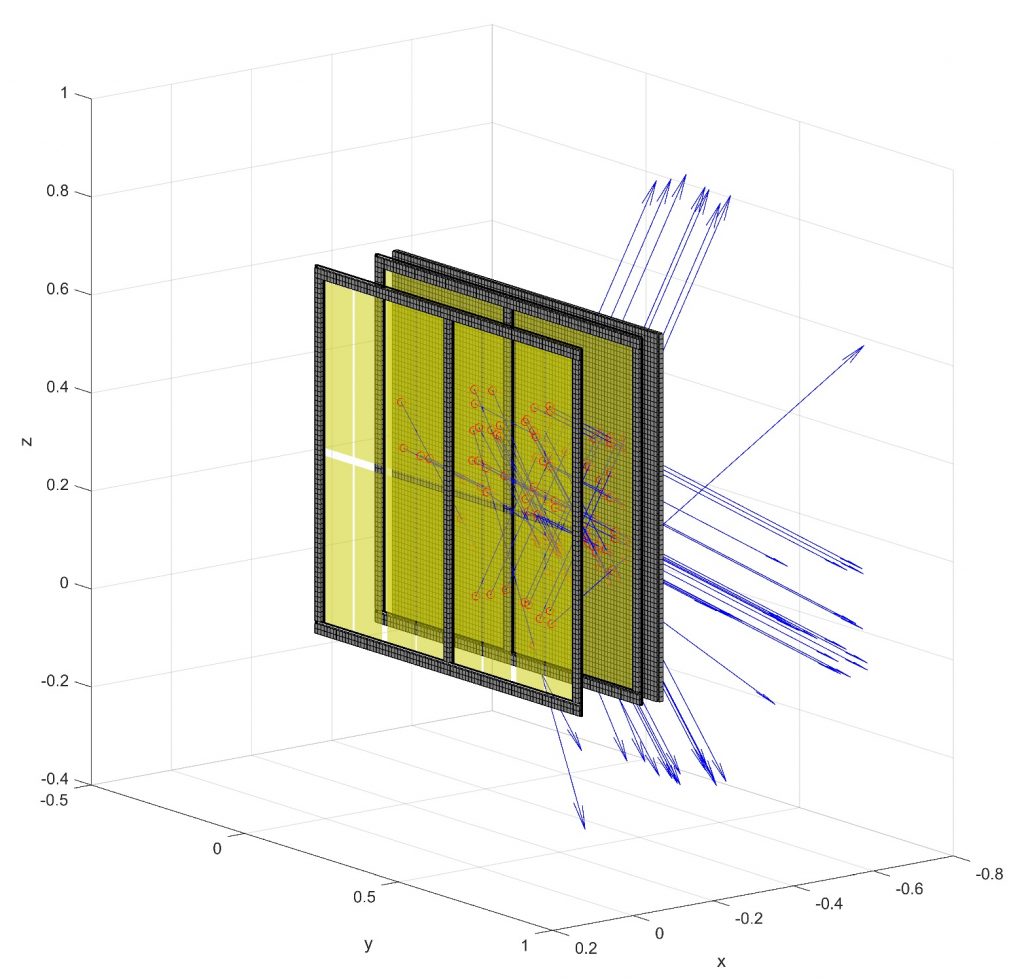