Mars Orbiter Mission – Spacecraft & Mission
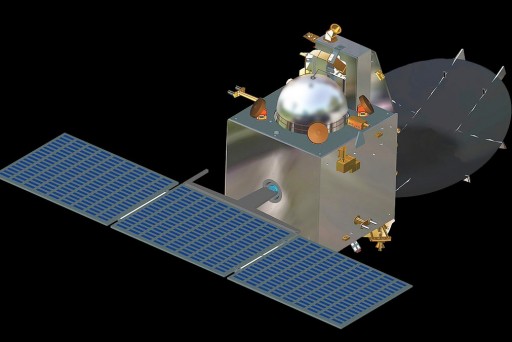
The Mars Orbiter Mission MOM, sometimes called Mangalyaan, is India’s first mission to Mars set for launch aboard a Polar Satellite Launch Vehicle in November 2013 for an arrival at Mars in 2014. The 1,337-Kilogram spacecraft carries a suite of five instruments to study Mars, its atmosphere and acquire photos of the Red Planet. Most importantly, the mission serves as a demonstration mission with the main objective of placing Mangalyaan in orbit around Mars as a study for future spacecraft and mission design.
The mission was put together on rather short notice – being approved in August 2012 with just 15 months to go until the Interplanetary Launch window that comes once every 26 months. The development of the mission was initiated one year earlier.
The Mars Orbiter Mission was approved by the Indian Government after the Indian Space Research Organization completed a project study.
Mangalyaan was approved for a total project cost of $69 million. In 2012, the individual components of the orbiter began assembly before the spacecraft came together in March 2013. The instruments started integration with the spacecraft in April to begin testing in August and September without much margin of error for meeting the launch window that stretches from October 28, 2013 to November 19, 2013.
The Spacecraft
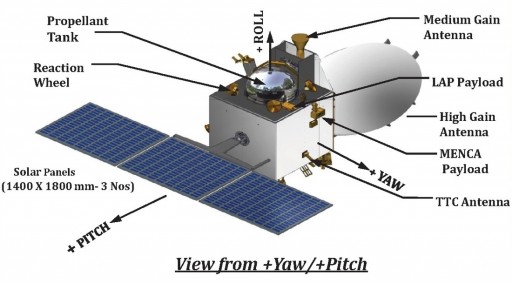
The Mars Orbiter Mission spacecraft is largely based on the Chandrayaan-1 Moon Orbiter featuring the same core structure and spacecraft systems. The Mangalyaan spacecraft bus is cuboid in shape featuring composite and metallic honeycomb sandwich panels and a central composite cylinder that facilitates all spacecraft equipment that is mounted on the panels as well as the cylinder. Fully integrated and fueled for flight, Mangalyaan weighs in at 1,337 Kilograms. The spacecraft has a dry mass of 475 Kilograms including a payload mass of 15 Kilograms and it carries a fuel load of 852 Kilograms.
The spacecraft is equipped with a single deployable solar array that consists of three panels – each being 1.4 by 1.8 meters in size. The assembly also includes a yoke and drive mechanism. The solar array provides 840 Watts of electrical power at Mars that is fed to a power distribution unit that provides power to the various systems and payloads and controls the state of charge of a 36-Amp-hour battery for night passes.
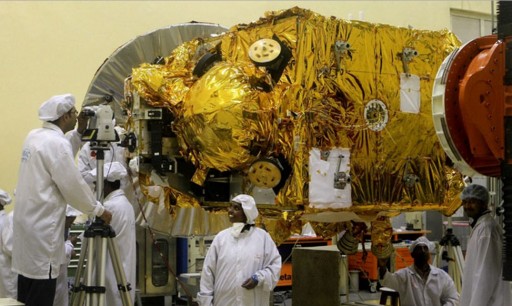
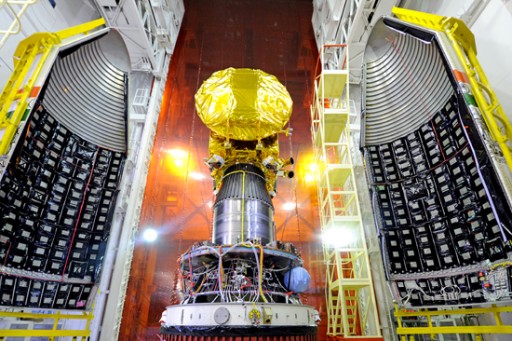
Mangalyaan is equipped with a bipropellant Main Propulsion System and an Attitude Control System. The Propulsion System features two spherical propellant tanks each holding 390 liters of propellant. Mangalyaan uses Unsymmetrical Dimethylhydrazine as fuel and Mixed Oxides of Nitrogen [MON-3: Nitrogen Tetroxide with 3% Nitric Oxide] as oxidizer that is fed to the engines via propellant lines and latch valves as pressure regulators. Tank pressurization is accomplished with high-pressure Helium. The He is stored in tanks at a pressure of 23.5Mpa that is regulated down to under 2MPa for tank pressurization.
The Main Propulsion System is centered around the Liquid Apogee Motor which has become the Indian workhorse on Geostationary Satellites and its previous Moon probe. LAM has demonstrated its capabilities in space many times, but for Mangalyaan, it has to be ensured that the engine can still fire after a 300-day coast to Mars for the orbit insertion maneuver – which is required for mission success.
LAM provides 440 Newtons of thrust which equates to 44.87 Kilograms. The engine operates and an mixture ratio (O/F) of 1.65 and has a nozzle ratio of 160 providing a specific impulse of 3,041N*sec/kg. The engine’s injector is a co-axial swirl element made of titanium while the thrust chamber is constructed of Columbium alloy that is radiatively cooled. Electron welding technique is used to mate the injector to the combustion chamber.
LAM is a robust engine that can tolerate injection pressures of 0.9 to 2.0 MPa, propellant temperatures of 0 to 65°C, mixture ratios of 1.2 to 2.0 and bus voltages of 28 to 42 Volts. The engine is certified for long firings of up to 3,000 seconds and a cumulative firing time of >23,542 seconds.
MOM is equipped with a propulsive Attitude Control System consisting of eight 22-Newton thrusters that also use UMDH and MON-3 propellants.
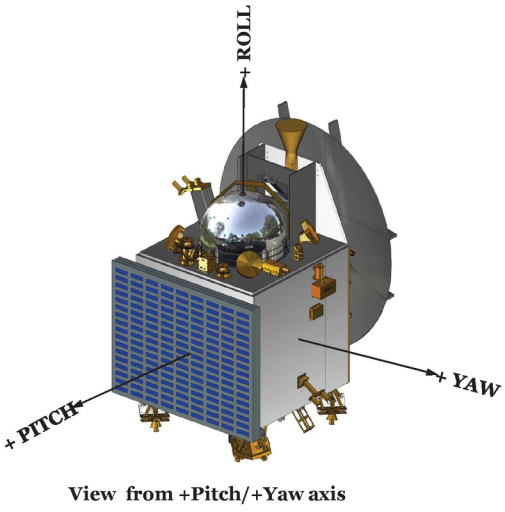
The thruster also uses a co-axial swirl type Titanium alloy injector and a Columbium combustion chamber. The thrusters operate in blowdown mode at a chamber pressure of 0.68 Mpa creating a specific impulse of 2,780 N*sec/kg. The 22N thrusters have an area ratio of 100. It can be operated in pulse mode with a minimum pulse duration of 8 milliseconds that supplies a minimum impulse of 65mN*sec. Each 22N thruster assembly weighs 0.8 Kilograms.
The 22N thruster is qualified for 300,000 duty cycles as it is mostly operated in pulse mode, but it can also withstand a single burn of up to 10,000 seconds and a cumulative burn time of 70,000 seconds. The engine tolerates a variety of operating conditions: 0.9 to 1.9 MPa on injection pressure, 0.2 to 2.0 on mixture ratio, -5 to 65°C on prop temperature and 28 to 42 Volts on bus voltage.
In addition to a propulsive Attitude Control System, the MOM spacecraft is equipped with four reaction wheels. Attitude and navigation data is provided by two star trackers and gyros as well as a coarse Sun sensor with nine heads. Attitude data is also provided by an Inertial Reference Unit and Accelerometer Package. The spacecraft features a dual redundant bus management unit for attitude control and command processing and execution. The Attitude and Orbit Control Electronics are centered around a MAR31750 processor.
Mangalyaan is equipped with a 2.2-meter diameter High Gain Antenna which is a parabolic X-Band reflector antenna that is used for data downlink and command uplink. Science data and spacecraft telemetry is stored in two 16Gb Solid State Recorders aboard the vehicle for downlink during regular communications sessions. Low and Medium Gain Antennas are used for low-bandwidth communications such as command uplink and systems telemetry downlink.
Science Instruments
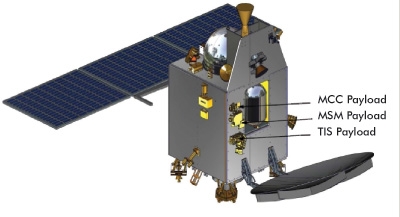
Mangalyaan carries a 15-Kilogram payload suite that consists of five scientific instruments:
- Lyman Alpha Photometer – LAP
- Martian Exospheric Neutral Composition Analyzer – MENCA
- Mars Color Camera – MCC
- Methane Sensor for Mars – MSM
- Thermal Infrared Imaging System – TIS
Lyman Alpha Photometer – LAP
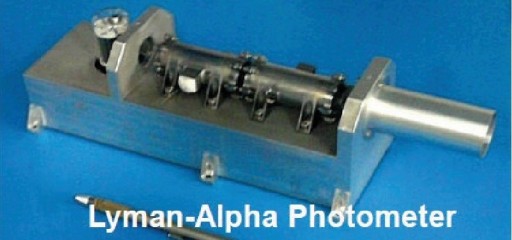
The Lyman Alpha Photometer payload consists of an Ultraviolet Detector that is equipped with gas-filled pure molecular hydrogen and deuterium cells with tungsten filaments that are located between an objective assembly and the UV detector.
LAP weighs about 1.5 Kilograms. Its core objective is the precise measurement of the Deuterium-to-Hydrogen abundance ratio which is an indicator for atmospheric loss processes and the role of Water in these processes.
Martian Exospheric Neutral Composition Analyzer – MENCA
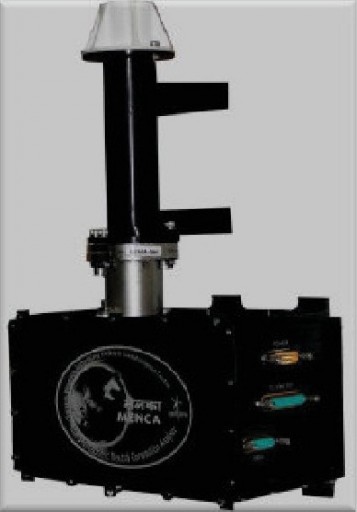
The heart of the 4-Kilogram MENCA payload is a quadrupole mass spectrometer. A Quadrupole Mass Spectrometer consists of four parallel metal rods with opposing rods being connected electrically. A radio frequency voltage is applied between the two pairs of rods and a direct current voltage is then superimposed on the RF voltage. Ions entering the instrument travel down the quadrupole between the rods.
Depending on their mass-to-charge ratio, ions either enter unstable trajectories and collide with the rods or make it through to the detector. The m/z of ions reaching the detector is a function of the voltage setting which allows the operator to select an ion with a particular mass-to-charge ratio to measure its abundance or run the instrument through a range of voltages to scan for a number of species that might be present.
Ions are generated via electron ionization. Electrons are produced through thermionic emission. The electrons are accelerated in an electric field and focused into a beam by a trap electrode. The atoms and molecules enter the ion source perpendicular to the electron beam. As high-energy electrons pass by and collide with the particles, large fluctuations in the electric field around the neutral molecules are caused leading to ionization and fragmentation.
The MENCA instrument operates at an m/z range of 1 to 300 u (atomic mass unit) with a mass resolution of 0.5u which allows detailed detection of species. The instrument can operate at the low partial pressure found in the upper Martian atmosphere.
The core objective of MENCA is to study the exospheric neutral density and composition at altitudes as low as 372 Kilometers above the Martian surface. The instrument examines radial, diurnal, and seasonal variations in the Martian exosphere. With Mangalyaan in its operational orbit, the spacecraft will have close encounters with Mars Moon Phobos which allows MENCA to estimate the upper limits of the neutral density distribution and composition around it. Studying the Martian exosphere will provide valuable data on the present conditions as well as atmospheric loss phenomena.
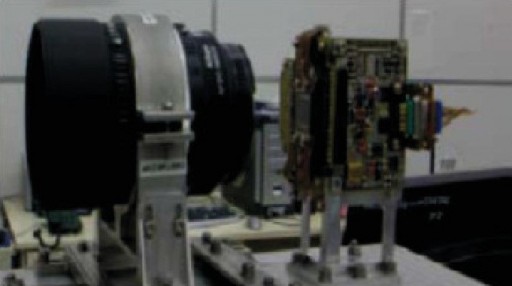
Mars Color Camera – MCC
Mangalyaan carries a camera payload that acquires color images of planet Mars. The payload weighs about 1.4 Kilograms. MCC covers a spectral range of 400 to 700 nanometers – the visible spectrum. The camera includes a multi-element lens assembly and a 2,000 by 2,000-pixel array detector with RGB Bayer Filter.
At periapsis, MCC provides images with a 50 by 50-Kilometer frame size and a resolution of 25 meters per pixel. At apoapsis, the camera provides a wide field of view of 8,000 by 8,000 Kilometers. Imagery provided by MCC are used to study Martian surface topography.
Methane Sensor for Mars – MSM
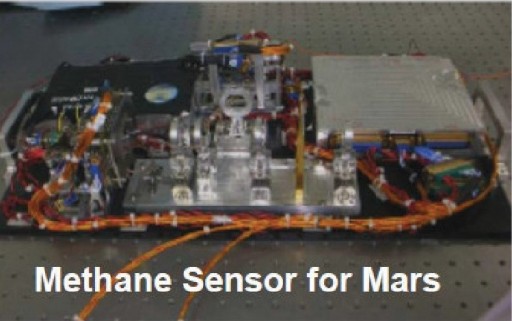
The MSM Payload weighs 3.6 Kilograms and is designed to measure methane concentrations in the Martian atmosphere with parts per billion accuracy. The instrument features a Fabry-Perot Etalon sensor that uses multiple beam interferometry at wavelengths of 1,642 to 1,658 nanometers to detect the signature of Methane that has a characteristic overtone band at these wavelengths.
The Fabry-Pérot-Etalon is comprised of an optical resonator consisting of a single plate with two parallel reflecting surfaces. Light passing into the instrument can only pass through the system when its wavelength corresponds to the resonances of the etalon that creates a narrow-band spectrum on the focal plane.
Thermal Infrared Imaging Spectrometer – TIS
The TIS instrument weighs 4 Kilograms and consists of a spectrometer that features a typical infrared grating spectrometer design. In the common design, radiation is directed through an entrance slit (available light energy depends on light intensity of the source as well as the dimensions of the slit and acceptance angle of the system). The slit is placed at the effective focus of a collimator that directs collimated radiation (focused at infinity) to a diffraction grating that acts as dispersive element. Another mirror refocuses the dispersed radiation onto a detector.
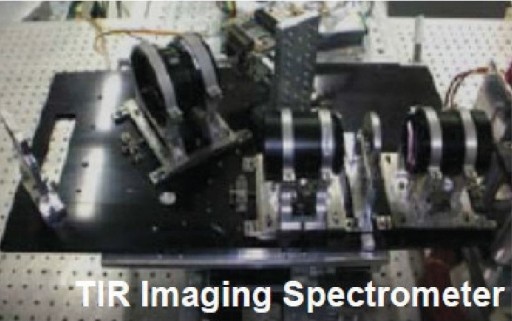
TIS uses a 120 by 160 element bolometer array detector. Bolometer receivers measure the energy of incoming photons. TIS is sensitive for an infrared wavelength range of 7 to 14 microns.
The microbolometer array does not require cooling. Each pixel on the array consists of several layers including an infrared absorbing material and a reflector underneath it that directs IR radiation that passes through the absorber back to the absorbing layer to ensure a near complete absorption. As IR radiation strikes the detector, the absorbing material is heated and changes its electrical resistance which can be measured via electrodes connected to each microbolometer and processed into an intensity read-out in order to create an IR spectrum.
The TIS instrument measures thermal emissions from the Martian surface to deduce surface composition and mineralogy.
Mission Design
Launch & Insertion
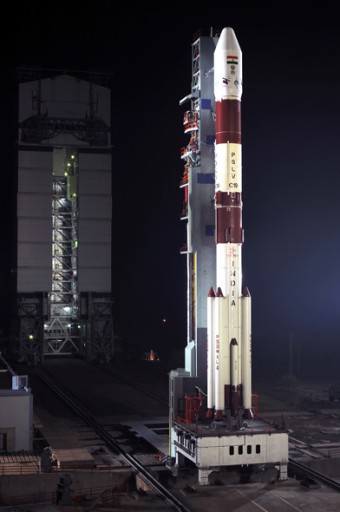
The Mars Orbiter Mission is planned to launch on an Indian Polar Satellite Launch Vehicle flying in its XL configuration. Mangalyaan does not use a direct injection in which the launch vehicle delivers the spacecraft to its Trans-Martian Trajectory. Instead, Mangalyaan is delivered to Earth orbit from where it uses its own propulsion system to insert itself into its TMI trajectory over a period of weeks. This design still requires the spacecraft to be launched within a narrow window that is only open for a few days every 26 months. The MOM launch window opens on October 28, 2013 and extends through November 19, 2013.
The Polar Satellite Launch Vehicle in its XL Version stands 44.5 meters tall, has a core diameter of 2.8 meters and a liftoff mass of 320,000 Kilograms. It is a four-stage rocket that uses a combination of solid rocket stages and liquid-fueled stages. The launcher can deliver payloads of up to 1,410 Kilograms to Geosynchronous Transfer Orbit and is not capable of delivering payloads of this weight-class to interplanetary trajectories – requiring a different approach to Lunar or Mars missions using PSLV.
The PSLV launcher consists of a large core stage that is 20.34 meters long and holds 138,000 Kilograms of solid propellant – making it one of the largest solid rocket stages ever flown. It provides a whopping thrust of 495,600 Kilograms. Clustered around the core are six Solid Rocket Boosters – each being 1 meter in diameter and 13.5 meters long holding 12,000 Kilograms of propellant. Each of the boosters provides 51,250 Kilograms of thrust.
The second stage of the launch vehicle uses storable propellants, Unsymmetrical Dimethylhydrazine fuel and Nitrogen Tetroxide oxidizer, that are consumed by a single Vikas 4 engine that provides 81,500kg of vacuum thrust. The stage is 12.8 meters long featuring a 40,700-Kilogram propellant load.
The PS3 stage of the PSLV launcher is solid-fueled, being 2.02 meters in diameter and 3.54 meters long holding 6,700 Kilograms of HTPB-based propellant. The third stage provides a total thrust of 24,900 Kilograms. Stacked atop the third stage is the PS4 Upper Stage that again uses hypergolic propellants – Monomethylhydrazine fuel and Mixed Oxides of Nitrogen – consumed by two L-2-5 engines. The stage is 2.02 meters in diameter and 2.6 meters long featuring a fuel load of 2,920 Kilograms. Upper stage thrust is 1,500 Kilograms.
Launch Sequence
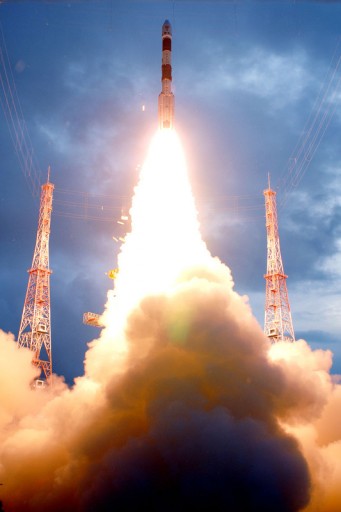
Following the completion of a 56.5-hour countdown, PSLV will launch with the Mangalyaan spacecraft hidden under its 3.2 by 8.3-meter payload fairing. The rocket will basically follow a standard mission profile to a Geosynchronous Transfer Orbit type orbit from where the MOM spacecraft begins its long journey to Mars. PSLV launches from the Satish Dhawan Space Center located on India’s East Coast.
At the moment of T-0, the PS1 Stage is ignited followed 0.5 seconds later by Boosters 1&2 and another 0.2 seconds later by Boosters 3&4 to create a total launch thrust of 700,600 Kilograms. Blasting off at a thrust to weight ratio of 2.18, initial ascent is very quick as PSLV races into the sky, starting a Pitch and Roll maneuver to align itself with a pre-planned ascent trajectory taking it south-east across the Indian Ocean.
The remaining Boosters (5&6) are ignited at T+25 seconds when the vehicle is already 2.5 Kilometers in altitude. Each of the Boosters burns for 49.5 seconds. The four ground-lit boosters are separated at T+1 minute and 10 seconds and fall into the Ocean. The air-lit boosters are jettisoned 22 seconds later enabling the PS1 stage to continue ascent on its own.
Throughout the booster phase and PS1 burn, three axis control is provided by a Secondary Injection Thrust Vector Control (SITVC) for yaw and pitch and two radially mounted thrusters for roll.
When the first stage has burned out, it separates from the second stage at T+1:53 followed by PS2 ignition an instant later. Staging occurs at approximately 58 Kilometers in altitude. During the second stage burn, the launch vehicle departs the dense atmosphere – allowing the vehicle to jettison its payload fairing at T+3:22 at an altitude of 113 Kilometers, exposing the MOM spacecraft for the remainder of the ascent as aerodynamic forces can no longer damage the vehicle. Vehicle control during second stage flight is provided by engine gimbaling for pitch and yaw and a roll reaction control system.
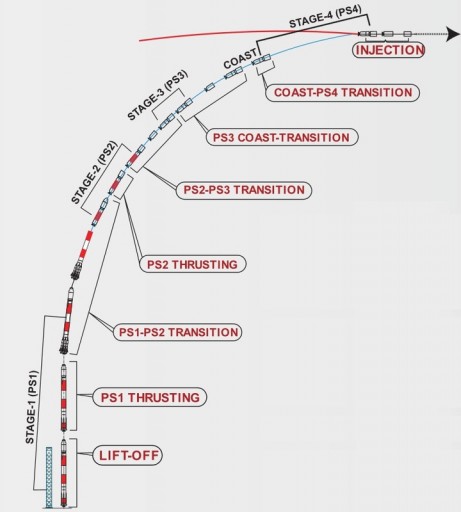
The second stage burns for about two minutes and 35 seconds before separating from the third stage that then ignites and assumes control of the flight at T+4:26. The solid-fueled third stage burns for 112 seconds to boost the stack to a sub-orbital trajectory. It uses the fourth stage Reaction Control System for three-axis control. After burnout of the PS3 stage, the stack begins a coast phase – initially holding onto the spent third stage before separating it at T+9:43 and continuing to coast uphill.
This coast phase allows the vehicle to fly uphill so that the fourth stage burn can increase the apogee altitude and also put a few Kilometers onto the perigee to place the stack in a stable orbit. MOM is targeting a higher Argument of Perigee than all previous ISRO mission into GTO in order to minimize the energy required from the GTO-type orbit to the interplanetary trajectory. Therefore, the coast between the 3rd and 4th stage burns is extended to 25 minutes.
The long coasting necessitated specific modifications to the coast phase guidance algorithm, on-board battery capacity augmentation, assessment on the performance of inertial systems for extended flight duration and deployment of two tracking ships to acquire the critical telemetry data during 4th stage flight and MOM separation.
Once the stack reaches its desired altitude, the two L-2-5 engines of the fourth stage ignite at T+35 minutes on a burn of about 8.5 minutes to boost the stack into its Transfer Orbit.
The Mars Orbiter Mission targets an injection orbit of 250 by 23,500 Kilometers at an inclination of 19.2 degrees. MOM separation takes place 44 minutes and 16 seconds after launch.
Approximate Ground Track
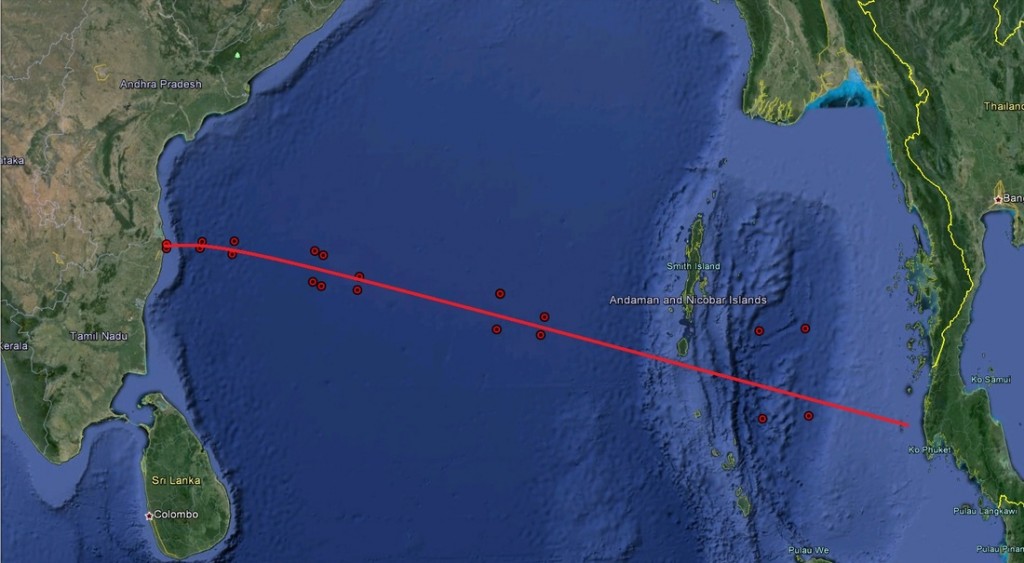
Trans-Martian Flight Profile
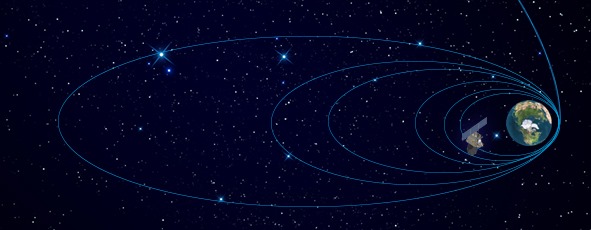
Being delivered to a 250 by 23,500-Kilometer orbit at an inclination of 19.2 degrees, the Mars Orbiter starts out in a type of GSO Transfer Orbit from where it will boost itself into a Trans-Martian Trajectory over a period of four weeks. MOM departs Earth orbit on November 30, 2013 .
This mission design was developed to accommodate the relatively low Payload Capability of the PSLV for an interplanetary mission. A similar profile was being utilized on the Chandrayaan-1 Moon Mission that launched in 2008.
Spending more than four weeks in Earth Orbit requires MOM to be equipped with radiation shielding to endure the numerous passages through Earth’s Radiation Belts.
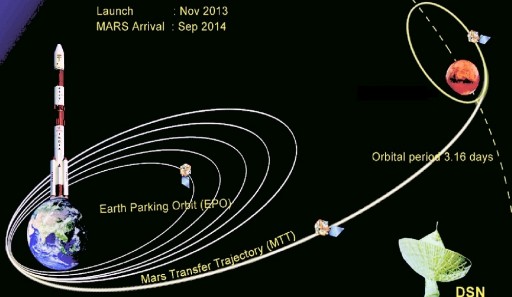
Over the course of its stay in Earth orbit, MOM fires its Liquid Apogee Motor six times – always when passing perigee to gradually increase the apogee of the orbit to work its way up to departing Earth orbit in a fuel-efficient manner.
The fifth firing places the spacecraft in a 600 by 215,000-Kilometer orbit around Earth and sets up the proper perigee passage for the final engine burn that puts the vehicle onto its Trans-Martian Trajectory.
The trip to Mars takes about 300 days and features a number of Trajectory Corrections. Shortly after TMI, when the precise trajectory has been determined, MOM corrects any insertion errors on its path to Mars. Later in the mission, when the vehicle is approaching the Red Planet, more Trajectory Correction Maneuvers are performed to target the proper position for the important Mars Orbit Insertion Maneuver.
Assuming and on-time launch and Earth Orbit Departure, Mangalyaan reaches Mars on September 24, 2014.
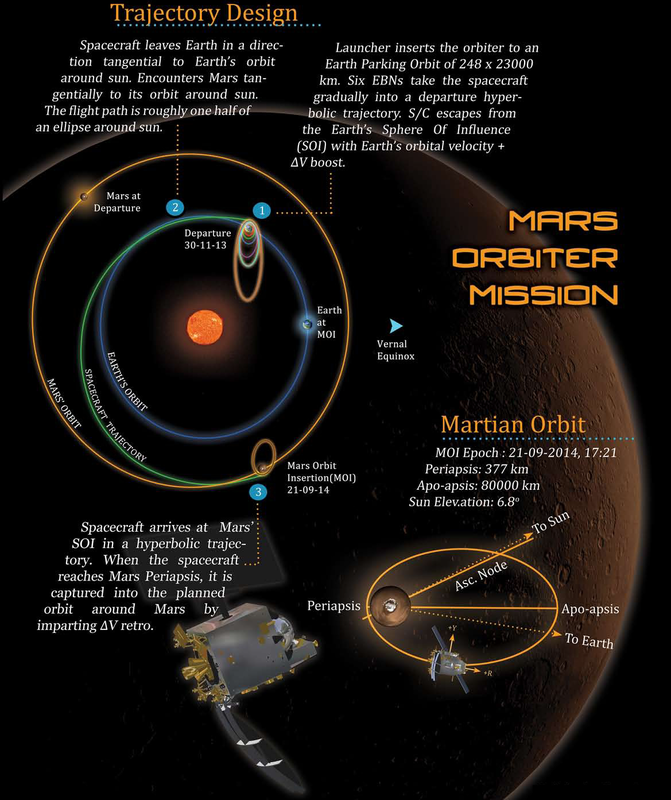
Mars Orbit & Science Mission
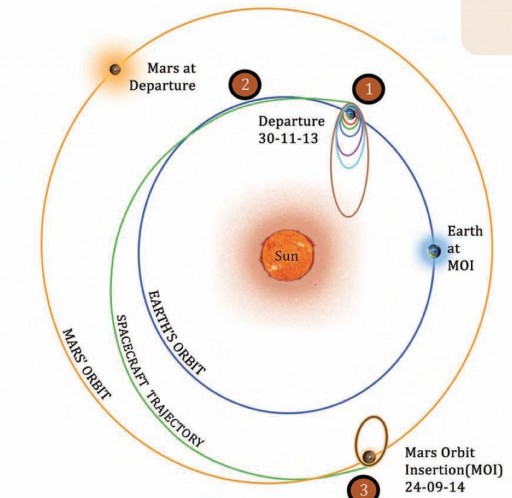
Arriving at Mars, Mangalyaan fires its Liquid Apogee Motor in order to insert itself into an elliptical orbit around Mars. The MOI Maneuver is the biggest nail-biter of the mission as the Liquid Apogee Motor has to function properly even after spending months in cruise mode to Mars.
Although LAM has flown on various GEO satellites and the Chandrayaan-1 Moon Probe, the engine has never had such a long break in between duty cycles performed in space. Should the MOI Burn not start on time or achieve the required change in velocity, Mangalyaan would be lost in its orbit around the sun.
The Mars Orbiter targets an operational orbit of 365.3 by 80,000 Kilometers with an inclination of 150 degrees and a duration of 76.72 hours from where it will perform its science mission. The MOM mission in Mars orbit is open-ended and is expected to last about 160 days.
Communications during the Mangalyaan mission are supported by India’s Deep Space Network Ground Stations while coverage for critical mission events such as Mars Orbit Insertion is supported by NASA’s global Deep Space Network.
MOM Objectives
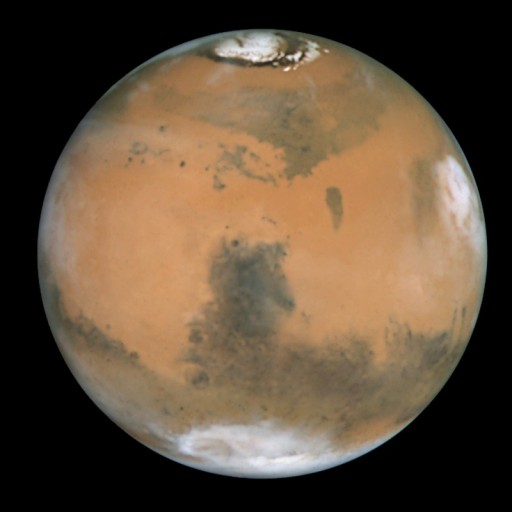
The specific objectives of the Mars Orbiter Mission are primarily associated with spacecraft construction and mission operations as Mangalyaan serves as a pathfinder, being India’s first mission beyond the Moon which brings its own unique challenges such as the 20-minute average signal delay to Mars. The Indian Space Science Data Center has provided the following Mission Objectives:
- Develop the technologies required for design, planning, management and operations of an interplanetary mission
- Orbit maneuvers to transfer the spacecraft from an elliptical Earth orbit to a heliocentric trajectory and finally insert it into Mars orbit
- Development of force models and algorithms for orbit and attitude computations and analyses
- Navigation in all mission phases
- Maintain the spacecraft in all phases of the Mission meeting Power, Communications, Thermal and Payload requirements
Incorporate autonomous features to handle contingency situations
The following scientific Objectives have been set for the Mars Orbiter Mission:
- Study climate, geology, origin and evolution of Mars
- To study sustainability of life on the planet
Mars Orbiter Mission – Science
The Mangalyaan Orbiter and its five instruments serve a number of scientific purposes to continue the exploration of Mars and its atmosphere. MOM will study surface mineralogy with its TIS instrument, continue the ongoing search for methane with its Methane Sensor, quantify the atmospheric loss on Mars, determine the neutral density of the Martian exosphere over an extended mass range and study the evolution of the atmosphere of the Red Planet.
Deuterium-Hydrogen Ratio as a Tracer of Atmospheric Loss
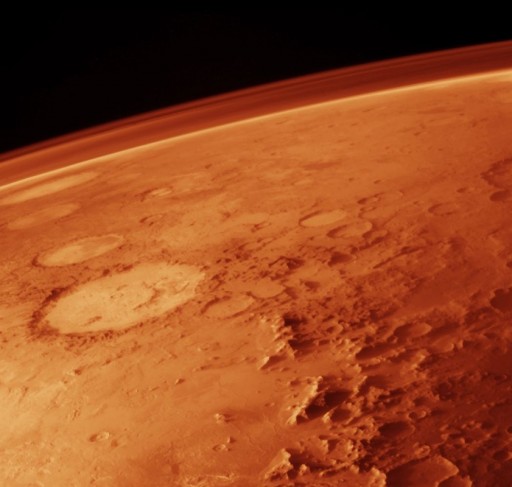
One item of special interest is the ratio of Deuterium and Hydrogen. Deuterium, also known as heavy hydrogen, is one of two stable isotopes of hydrogen, and contains one proton and one neutron in its nucleus while the common hydrogen isotope (Protium) has no neutron in the nucleus. Determining the D/H ratio in the atmosphere allows scientists to learn more about the atmospheric environments on Mars with a special focus on atmospheric loss.
Although the processes that might have caused atmospheric loss are not fully understood, it is known that if atmosphere is lost due to physical processes, lighter elements/isotopes are favored while heavier elements are retained because of gravity. This produces a difference in isotope abundances is over large time scales of hundreds of millions of billions of years.
Currently, scientists believe that the majority of the Martian Atmosphere was lost due to direct interaction of the atmosphere with the solar wind after Mars lost its protective magnetic field. This and other physical processes caused around 85 to 95% of the Martian Atmosphere to escape into deep space. The original atmosphere would have been warmer and it would have provided a substantially higher surface pressure which would have created an environment suitable for sustaining liquid water – a requirement for life as we know it.
Methane on Mars
Methane on Mars can have a number of origins. When methane was first discovered on Mars, many saw the presence of it as a clear indication of life or microbial activity, but aside from biological processes, methane can have a number of origins. Geological processes such as volcanism or reactions involving iron oxide, water and carbon dioxide can produce large amounts of the gas.
In addition to these two traditionally known origins on atmospheric methane, both, on Earth and on Mars, scientists have thrown a number of other theories on the table. In recent years, exogenous sources have been studied. Scientists at the Max Planck Institute for Chemistry in Mainz, Germany, and the universities in Utrecht and Edinburgh have published a theory on methane being produced by micro-meteorites and interplanetary dust particles that enter the thin Martian atmosphere and settle on the surface.
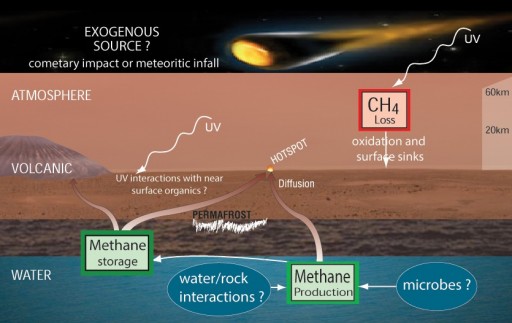
The researches have used meteorites that were found on Earth’s surface and subjected those to conditions identical to those encountered on the surface of Mars. What they found was a large amount of methane being released by the meteorites under the influence of UV radiation levels that are present on Mars.
Another source of atmospheric Methane could be Martian Dust Devils, as a team of scientists at the Metropolitan Autonomous University, Azcapotzalco, Mexico found: “We propose a new production mechanism for methane based on the effect of electrical discharges over iced surfaces. The discharges, caused by electrification of dust devils and sand storms, ionize gaseous CO2 and water molecules and their byproducts recombine to produce methane.” (A. Robledo-Martinez et al., Electrical discharges as a possible source of methane on Mars: Lab simulation, 2012)
Under the conditions on Mars, methane would quickly break down due to ultraviolet radiation coming from the Sun, interactions of the gas with the surface such as sinkage and oxidation, and chemical reactions with other gases in the Martian atmosphere, so for a permanent presence of methane on Mars, a continuous source would have to be present to replenish the atmospheric methane.
The processes of possible Methane storage and production of Mars as well as Methane destruction are not yet understood. In addition, the question whether methane, if present, is created by biological or geological processes, is still to be answered.
Past Methane Detections
The debate about methane on Mars started way back in 1969 when scientists working with the Mariner 7 Spacecraft announced that the spacecraft had likely detected Methane with its Infrared Spectrometer Instrument. This announcement was made just 48 hours after receiving data from the Spacecraft that recorded Infrared Spectra while performing its flyby of Mars. The spectra included bands that were not associated with carbon dioxide or other known substances on Mars and, after briefly performing lab tests, the team jumped to the conclusion of methane being present on Mars.
The announcement created a huge stir because of its implications to life on Mars, however, project scientists publicly retracted the finding several weeks later after completing additional analysis of the spectra acquired by Mariner 7 that revealed that the unexpected band at a wavelength of 3.3μm that was mistaken for methane was actually created by carbon dioxide ice in an unexpected configuration.
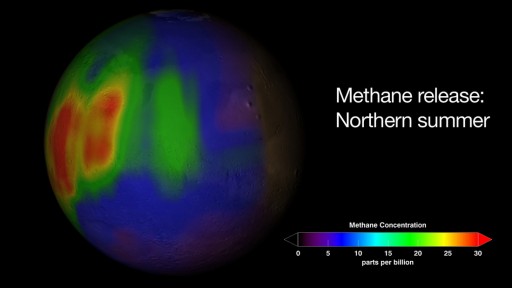
Several decades later, in 2003, a team at NASA’s Goddard Space Flight Center that was studying the Martian atmosphere, announced that trace amounts of methane at the level of several parts per billion were found to be present in the atmosphere of the planet. In 2004, data from ESA’s Mars Express Orbiter and ground-based telescope observations suggested even higher quantities of methane of up to 10ppb.
Data acquired by telescopes and Mars orbiters have led to new theories as a large variability of atmospheric methane in time and space was suggested. In a paper released in 2009, Michael Mumma of NASA’s Goddard Space Flight Center presented a hotspot theory based on data acquired in 2003 and 2006. “Methane is quickly destroyed in the Martian atmosphere in a variety of ways, so our discovery of substantial plumes of methane in the northern hemisphere of Mars in 2003 indicates some ongoing process is releasing the gas,” he said in 2009.
“When present, methane occurred in extended plumes and the maxima of latitudinal profiles imply that the methane was released from discrete regions. At northern mid-summer, the principal plume contained ~19,000 metric tons of methane.” (Mumma et al., Strong Release of Methane on Mars in Northern Summer 2003; 2009) The localized hotspot peaked at about 60ppb, before the methane concentration started to decrease. The lifetime of the hotspot was under one year. Whether the methane plume was biological or geological in origin is unknown. “Right now, we don’t have enough information to tell if biology or geology — or both — is producing the methane on Mars,” said Mumma (2009). “But it does tell us that the planet is still alive, at least in a geologic sense. It’s as if Mars is challenging us, saying, hey, find out what this means.”